2 Life Cycle Optimization Framework for Pump and Treat Systems
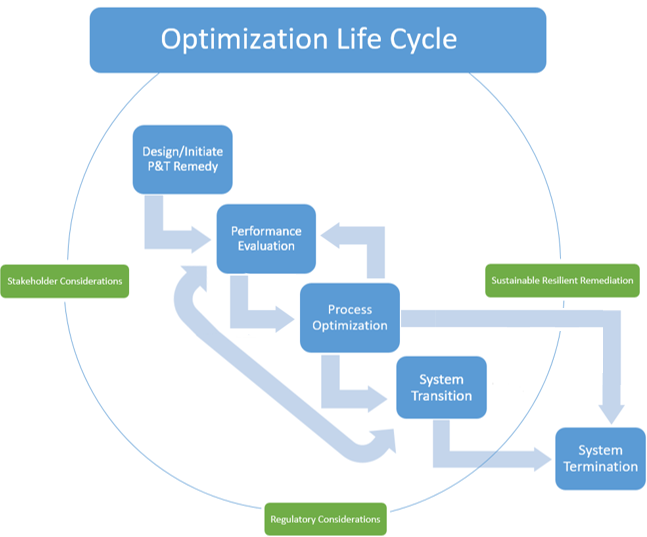
Optimization Life Cycle Navigation Diagram
Source: E. Madden, ITRC. Used with Permission.
This section provides a framework for conducting optimization during the life cycle of a groundwater P&T system. Figure 2‑1 below depicts the conceptual site model (CSM) life cycle from initial site assessment through design, implementation, O&M, and remedy transitioning or site completion. Within the context of performance-based optimization, the focus of this guidance document is on remedy implementation, O&M, and transitioning or site completion.
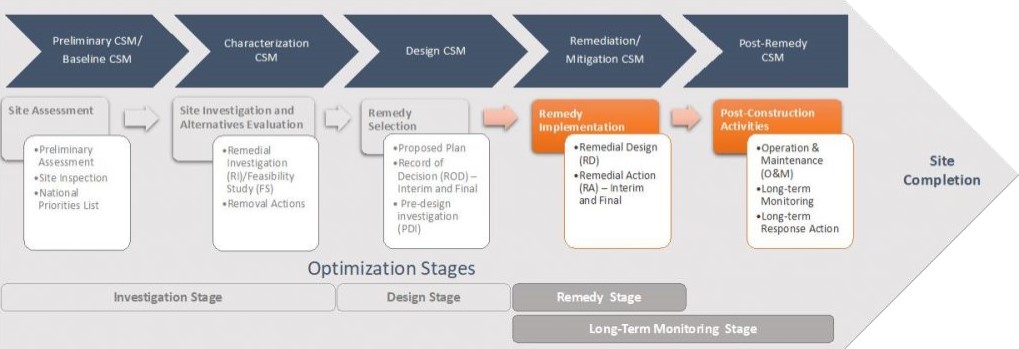
Adapted from ( USEPA 2012[ENEAAYBS] USEPA. 2012. “National Strategy to Expand Superfund Optimization Practices from Site Assessment to Site Completion.” U.S. Environmental Protection Agency, Office of Solid Waste and Emergency Response. https://nepis.epa.gov/Exe/ZyPURL.cgi?Dockey=P100GI85.txt. ).
Remedy optimization is an important component of a life cycle approach to identifying and implementing optimization practices for cleanup efforts. Remedy optimization applies to the entire life cycle, from site assessment efforts through site completion. Optimization in general is defined as efforts at any phase of a removal or remedial response to identify and implement specific actions to improve the effectiveness and cost-efficiency of the remedy during that phase ( USEPA 2012[ENEAAYBS] USEPA. 2012. “National Strategy to Expand Superfund Optimization Practices from Site Assessment to Site Completion.” U.S. Environmental Protection Agency, Office of Solid Waste and Emergency Response. https://nepis.epa.gov/Exe/ZyPURL.cgi?Dockey=P100GI85.txt. ). As discussed throughout this section, optimization of P&T remedies can provide significant benefits such as accelerating cleanup and reducing the active time frame to achieve the goals of the remedy. Optimization can be conducted periodically based on performance assessments and additional CSM information, while considering the remedy goals (as discussed in more detail in Section 2.2). These optimization efforts may address issues such as protectiveness concerns, high uncertainties or data gaps associated with CSMs, technological challenges, maintaining effectiveness and efficiency of the cleanup approach, high costs associated with O&M, and unattainable remedy objectives (e.g., remedial action objectives (RAOs)). Therefore, optimization efforts are expected to maintain or improve the remedy’s protectiveness and long-term implementation, which may accelerate progress toward site completion and/or transitioning.
The framework discussed in this section relies on information gathered from existing optimization programs developed by various private, state, and federal agencies (e.g., USEPA, United States Department of Defense (DOD)) for cleanup activities. Some of the common elements of remedy optimization in these programs include site characterization and remedial investigation (RI), independent design reviews, O&M of remedial systems (including remedial system evaluation (RSE)), and long-term monitoring optimization (LTMO). These programs and elements and their components relevant to P&T remedies are discussed in more detail in Section 2.1 and in Appendix D.
Subsection 2.3 provides a description of the interactive tool that supports decision-makers when they need to determine whether an optimization effort may be warranted at a given site. The interactive tool is intended to help users assess the performance of the remediation system and identify any optimization needs and to guide the users throughout this document to the appropriate section for additional information.
2.1 Pump and Treat Remedy Life Cycle
P&T systems have been selected as components of remedies for various types of contaminated sites (Superfund, RCRA, state programs such as landfills, petroleum) over the past several decades. P&T technology represents about 20% of the groundwater remedies selected during the 2015–2017 period under the Superfund program ( USEPA 2020[RLVPF7D5] USEPA. 2020. “Superfund Optimization Progress Report.” U.S. Environmental Protection Agency, Office of Land and Emergency Management. https://semspub.epa.gov/work/HQ/100002585.pdf. ). This represents a significant decline in the selection of P&T since the early 1980s, but it still highlights the importance of these remedies in cleanup efforts. P&T systems have been applied to (1) hydraulic containment and/or to a targeted portion of a plume (e.g., source area), (2) source remediation, and (3) removal of contaminant mass to make progress toward aquifer restoration. They can also be components of other remediation strategies involving in situ operations and treatments, such as lowering groundwater levels in support of soil vapor extraction ( SVE), multi-phase extraction systems (MPE), engineered bioremediation, in situ chemical oxidation (ISCO) or in situ chemical reduction (ISCR) efforts, or permeable reactive barriers (PRBs). In such cases, optimization of a P&T remedy must also consider the effects on other technologies being used in combination with P&T. It is important to consider the life cycle of P&T projects since P&T often represents a significant amount of the ongoing operational costs of installed remediation systems.
The main components of a P&T system (Figure 2‑2) typically include the following:
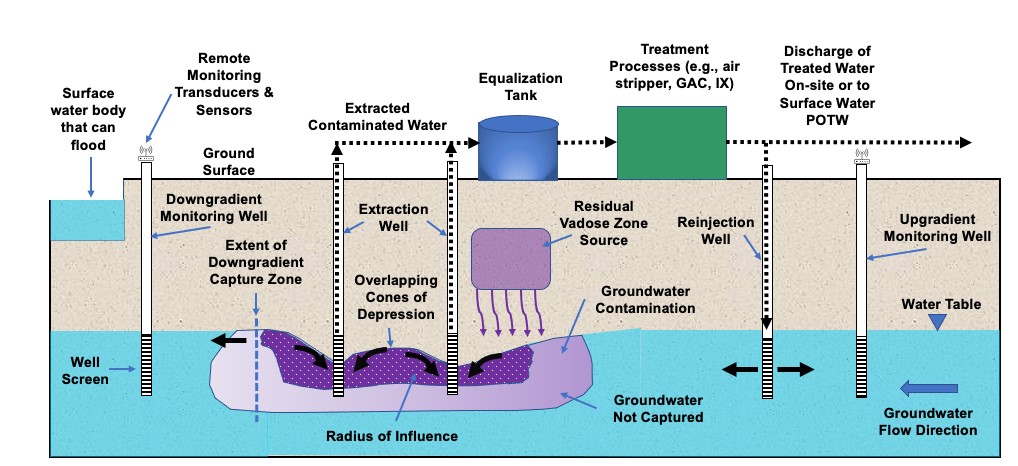
Adapted from https://frtr.gov/matrix/Groundwater-Pump-and-Treat/.
Within the Superfund program, system monitoring is not considered a component of a remedy; however, it is recognized that process and performance monitoring is integral to remedy implementation and a critical component of maintaining remedy effectiveness for P&T systems ( USEPA 2012[ENEAAYBS] USEPA. 2012. “National Strategy to Expand Superfund Optimization Practices from Site Assessment to Site Completion.” U.S. Environmental Protection Agency, Office of Solid Waste and Emergency Response. https://nepis.epa.gov/Exe/ZyPURL.cgi?Dockey=P100GI85.txt. ).
At many contaminated sites using P&T, early response actions were needed to focus on mitigating immediate risks to receptors while there was still significant uncertainty associated with the site CSMs. As a result, the initial design of P&T systems frequently focused on large-scale containment and bulk treatment. Those extraction systems were often not optimally configured for mass removal, and performance of those systems was impacted by uncertainties associated with complex factors such as heterogeneous geology, large capture zones requiring multiple pore volume flushes, the presence of source zones, diffusion-limited mass transfer, colocated and/or recalcitrant contaminants, and dispersed contaminant distributions ( NRC 2013[26RFNQD2] NRC. 2013. Alternatives for Managing the Nation’s Complex Contaminated Groundwater Sites: Washington, D.C.: National Academies of Sciences, Engineering, and Medicine. https://doi.org/10.17226/14668.Guo and Brusseau 2017[RQR7EH83] Guo, Z.L., and M.L. Brusseau. 2017. “The Impact of Well-Field Configuration on Contaminant Mass Removal and Plume Persistence for Homogeneous Versus Layered Systems.” Hydrological Processes 31 (26): 4748–56. https://doi.org/10.1002/hyp.11393. ). As the remedy progresses from initial implementation through continued operation over time, contaminant removal effectiveness typically diminishes due to these complexities. This reduction in remedy effectiveness often hinders achievement of concentration or mass reduction–based performance objectives and can result in extended cleanup time frames that can span many decades ( USEPA 1996[LPDXYRP7] USEPA. 1996. “Pump and Treat Remediation: A Guide for Decision Makers and Practitioners.” U.S. Environmental Protection Agency, Office of Research and Development. https://frtr.gov/matrix/documents/Groundwater-Pump-and-Treat/1996-Pump-and-Treat%20Ground-Water-Remediation.PDF.USEPA 1997[G2ZRNPLQ] USEPA. 1997. “Ground Water Issue: Design Guidelines for Conventional Pump-and-Treat Systems.” ). Suboptimal operations (e.g., aboveground treatment processes that may limit groundwater extraction rates or inadequate well-network configurations) and design (e.g., overestimation of the groundwater capture zone) can contribute to plume persistence and limited effectiveness of P&T systems. Back diffusion of contaminant mass present in low permeability units may result in increases in dissolved-phase concentrations (commonly referred to as “rebound”) after shutdown of extraction wells following early reductions in plume concentrations (Figure 2‑3). As plume conditions and removal effectiveness evolve over the course of treatment, the primary challenge at these sites is to monitor performance and maintain effectiveness while managing the P&T system toward site completion or a transition to another remedial approach such as passive treatment or monitored natural attenuation (MNA) ( Truex et al. 2017[3HUEBEH4] Truex, Michael, Chris Johnson, Tamzen Macbeth, Dave Becker, Kira Lynch, Dominic Giaudrone, Aaron Frantz, and Hope Lee. 2017. “Performance Assessment of Pump-and-Treat Systems.” Groundwater Monitoring & Remediation 37 (3): 28–44. https://doi.org/https://doi.org/10.1111/gwmr.12218. ). P&T transition is discussed in detail in Section 5. In addition to maintaining effectiveness and efficiency, there may be an opportunity to optimize P&T systems to address emerging contaminants, changing cleanup levels, or site reuse conditions.
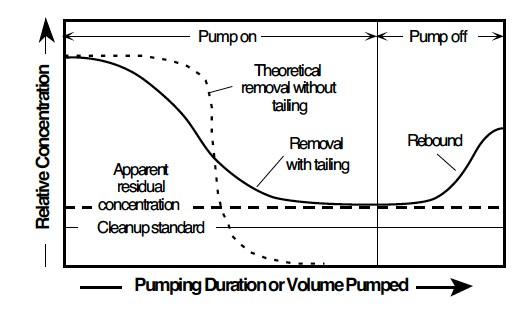
Adapted from USEPA 1994[NSKP8SN6] USEPA. 1994. “Methods for Monitoring Pump-and-Treat Performance.” https://semspub.epa.gov/work/HQ/174486.pdf. .
Remedy optimization is an important component of a life cycle approach for identifying and implementing optimization practices for cleanup efforts ( USEPA 2012[ENEAAYBS] USEPA. 2012. “National Strategy to Expand Superfund Optimization Practices from Site Assessment to Site Completion.” U.S. Environmental Protection Agency, Office of Solid Waste and Emergency Response. https://nepis.epa.gov/Exe/ZyPURL.cgi?Dockey=P100GI85.txt. ). Periodic optimization of P&T remedies can help maintain contaminant removal effectiveness throughout the remedy life cycle and move systems toward site completion or a treatment train transition strategy. For P&T systems, there are technical elements of optimization specific to the P&T technology that are needed to support an overall remedy optimization effort. A critical element for P&T performance optimization in relation to plume remediation efficiency and effectiveness is adapting the well-network approach (e.g., extraction well locations, well screen depth / well construction, and pumping rates) to evolving plume conditions as the remedy progresses. The well-network approach is a critical element that relies on monitoring and assessment of aquifer response and quality (see Section 3). Extraction, reinjection, and monitoring wells can comprise a well network and are all subject to optimization.
The following sections describe a performance-based optimization framework for P&T systems and the technical elements of optimization. Additionally, an interactive tool has been developed to provide guidance on identifying optimization needs for a given P&T system.
2.1.1 P&T Remedy Selection and Implementation
Containment and cleanup of contaminated groundwater are among the main objectives of CERCLA, RCRA, and state remediation programs ( Cohen et al. 1997[93N64ILB] Cohen, Robert M., James W. Mercer, Robert M. Greenwald, and Milovan S. Beljin. 1997. “Design Guidelines for Conventional Pump-and-Treat Systems.” U.S. Environmental Protection Agency. https://cfpub.epa.gov/si/si_public_record_report.cfm?Lab=NRMRL&dirEntryId=90422. ). P&T remedies for cleanup at sites with groundwater contamination are typically selected because they are intended to satisfy these two objectives.
Remedy selection under the Superfund program is approached through the remedial investigation/feasibility study (RI/FS) process, as provided in 40 Code of Federal Regulations (CFR) Part 300, and equivalent processes for the RI/FS process/responses exist within RCRA and state remediation programs. The National Oil and Hazardous Substances Pollution Contingency Plan (NCP) Subpart E provides the response process. Subpart F describes state involvement in hazardous substance response. Equivalent processes for the RI/FS process and responses exist within RCRA and state remediation programs. The RI process involves well-defined steps for the following: (1) collecting data to characterize site conditions, (2) determining the nature and extent of the contamination, and (3) assessing the risk to human health and the environment. The FS process serves as the mechanism for the development, screening, and detailed evaluation of alternative remedial actions, including treatability testing as necessary to evaluate the potential performance and cost of the treatment technologies that are being considered. ( USEPA 1998[Y4Q3F3Q8] USEPA. 1998. “Technical Protocol for Evaluating Natural Attenuation of Chlorinated Solvents in Ground Water.” https://clu-in.org/download/remed/protocol.pdf. ) These processes could be helpful for other hazardous and nonhazardous remedial programs.
Once a set of remedial alternatives has been developed, such as extraction of contaminated groundwater, the remedial action and applicable technologies for a specific site (e.g., extraction of contaminated groundwater) are selected based on a set of nine criteria and documented in a Proposed Plan and Record of Decision. These criteria include the following: (1) overall protection of human health and the environment; (2) compliance with applicable or relevant and appropriate requirements (ARARs) under federal or state laws); (3) long-term effectiveness and permanence; (4) reduction of toxicity, mobility, or volume through treatment; (5) short-term effectiveness (risks); (6) implementability; (7) cost; (8) state acceptance; and (9) community acceptance.
Choosing a P&T remedy is a function of desired remedy objectives ( USEPA 1994[NSKP8SN6] USEPA. 1994. “Methods for Monitoring Pump-and-Treat Performance.” https://semspub.epa.gov/work/HQ/174486.pdf. ). There are typically three basic categories of P&T implementation strategies, and the choice of strategy depends on the functional purpose and remedial goals, which can be (1) source control, (2) downgradient groundwater plume containment, and (3) downgradient groundwater plume mass reduction. The remedial strategy may include one or all of these approaches, and P&T is often used in combination with other remedial technologies to achieve site cleanup. The overall objective is to prevent or reduce the risks associated with the contaminated water (e.g., reaching a drinking water supply or entering a surface water body).
Source control refers to a design where the remedial goal is to intercept and capture high-concentration dissolved-phase contamination from the saturated zone adjacent to or within the source area. This approach removes mass from the saturated media in the source zone to avoid further contributions to downgradient plumes. Also, with source control, extraction wells are often situated to intercept the highest contaminant concentrations attenuating the total source mass, minimizing the required treatment capacity (i.e., flow-through capacity) in the P&T treatment unit. Not all sources at a given site may be identified in the CSM at the time of initial design; ongoing site characterization and/or remedy performance evaluations may reveal alternate sources that might need to be addressed through optimization. Additional contributions to downgradient plumes may become evident from performance monitoring and require further optimization of the system or measures to address newly identified sources. Where source control P&T operations are enhanced with additional remedies (e.g., vertical engineered groundwater barriers such as slurry walls and sheet pile), the optimization efforts would need to consider the hybrid systems.
Hydraulic plume containment requires a design where dissolved-phase contaminants are intercepted and removed at a specific location and depth to prevent or minimize continued expansion of the plume, or where feasible, to pull the plume back from expanded dimensions. This approach is sometimes selected where aquifer restoration is technically impracticable (e.g., due to back diffusion of contaminants, presence of subsurface nonaqueous-phase liquid (NAPL), large numbers of known and unknown legacy source areas, or other complicating conditions). Containment may also be needed to protect potential downgradient receptors or prevent migration of the plume beyond the site boundaries / geographic area ( USEPA 1994[NSKP8SN6] USEPA. 1994. “Methods for Monitoring Pump-and-Treat Performance.” https://semspub.epa.gov/work/HQ/174486.pdf. ). While this approach typically addresses an immediate risk, without the goal of mass removal (if mass removal is a potential for the aquifer, e.g., for large plumes with low migration rates and known sources) it is rarely implemented as a stand-alone remedy due to the lifetime costs for achieving remedial end-point goals. Where plume migration may take a long period of time, not addressing the plume early on with a mass removal and/or in situ treatment strategy may result in operating plume containment wells for a very long time and greater life cycle costs.
A plume mass reduction strategy for P&T systems focuses on removing mass from the plume with the goal of diminishing the dissolved-phase contamination below target concentrations (often drinking water standards under an aquifer restoration strategy). Mass concentrations within the plume can be reduced by P&T, usually through a dynamic approach of extraction-well network adjustments (e.g., well-network configuration changes, adjustment of pumping rates, etc.). Extraction wells are often located in the most contaminated portions of the plume to yield the greatest mass removal. Further, paired extraction and injection wells can be used to increase plume flushing to expedite plume remediation. Similar challenges arise when operating P&T systems for plume mass reduction, as they do for source control as described above. Dynamic hydrologic systems and evolving information about source areas can demonstrate a need for optimization to achieve cleanup goals.
At a complex site, a combination of source control, hydraulic plume containment, and plume reduction objectives should be considered to achieve cleanup goals. Detailed design of the P&T system should be directly impacted by the RAOs for the remedy, particularly regarding placement and operation of the extraction wells or trenches. In some cases, the initial design of the system may have addressed an interim remedial measure (IRM) based on the immediate risks for human health and the environment. These IRM systems may focus primarily on hydraulic containment of the migrating plumes and source control. While necessary, this approach may not yield the most effective contaminant mass recovery approach given that CSM uncertainties are the highest at the beginning of operations. Therefore, an IRM system may not be best positioned to achieve RAOs over the life cycle of the project. If cleanup is the ultimate objective, beyond immediate plume containment, performance monitoring and assessment will be crucial to understand system behavior during the implementation of a P&T remedy, to update the CSM, and to make modifications necessary to better target mass removal and/or other RAOs.
For example, under the Superfund program, the performance and monitoring assessments are usually conducted in conjunction with the formal Five Year Review process. Five Year Reviews are required to evaluate the implementation and performance of a remedy to determine whether it remains protective of human health and the environment ( USEPA 2001[PVUBSVVY] USEPA. 2001. “Comprehensive Five-Year Review Guidance.” U.S. Environmental Protection Agency, Office of Solid Waste and Emergency Response. https://semspub.epa.gov/work/HQ/128607.pdf. ). Technical assessments associated with these reviews evaluate whether the remedy is operating as designed and may identify opportunities for optimization of the system and groundwater monitoring activities or of the operating treatment systems themselves. In some cases, USEPA Regions may request optimization reviews in advance of, and in direct support of, completion of Five Year Review documents. In reality, P&T optimization, especially optimization of mass recovery or source control systems (e.g., extraction-well network), may be needed more frequently in response to performance monitoring and additional CSM information to maintain the effectiveness and efficiency of the remedy while also considering life cycle costs. Therefore, sole reliance on the Five Year Review process may not be appropriate at all sites to adequately optimize performance of a P&T system.
2.1.2 Stages of Assessment and Cleanup Processes (or Remedial Life Cycle) Relevant to Life Cycle Optimization of Pump and Treat Systems
Remedial actions comprise several phases or stages (see Figure 2‑1), which can be correlated with the P&T life cycle as summarized in Table 2‑1. The table presents the phases of the remedy life cycle, corresponding P&T life cycle stage, and associated typical P&T life cycle activities. The table incorporates the following P&T life cycle activities: (1) P&T selection and design, (2) implementation of the P&T system and determination that the system is functioning as designed through normal O&M and until the P&T system is either shutdown or transitioned to an alternate operational mode (e.g., hydraulic control only, adaptation for an in situ remedy), and (3) site completion.
Table 2‑1. P&T life cycle activities in relation to assessment and cleanup stages
Phases of Remedy Life Cycle |
Corresponding P&T Life Cycle Stage |
Associated Typical P&T Life Cycle Activities |
Site Assessment | Site assessment |
|
Site Investigation and Alternatives Evaluation | Remedial investigation |
|
Remedy Selection | Selection of P&T as a remedial technology |
|
Remedy Design | Design of the P&T system |
|
Remedy Implementation | Installation of the P&T system Start-up and early operation of the P&T system |
|
Post-Construction Activities | Ongoing system operation and monitoring Late life cycle operation Transition to system shutdown / MNA and/or alternate technologies |
|
Close-out and decommissioning of the P&T system |
|
Notes: COC = contaminant of concern, CSM = conceptual site model, MNA = monitored natural attenuation, O&M = operation and maintenance, P&T = Pump and Treat.
In addition to improving the short-term treatment effectiveness and cost-efficiency, optimization actions may also improve the remedy’s long-term protectiveness and cleanup efficiency, which may facilitate progress toward site completion. To identify these opportunities, a systematic site review is performed by a team of independent technical experts that applies a variety of techniques and approaches (e.g., green remediation and Triad ( USEPA 2011[B5FVZMT4] USEPA. 2011. “Environmental Cleanup Best Management Practices: Effective Use of the Project Life Cycle Conceptual Site Model.” Office of Solid Waste and Emergency Response. EPA 542-F-11-011. https://www.epa.gov/sites/default/files/2015-04/documents/csm-life-cycle-fact-sheet-final.pdf. ), Resource Conservation and Climate Resilience Screenings and Evaluations, Smart Scoping, high-resolution site characterization, and three-dimensional data visualization and analysis ( 3DVA)) to identify opportunities for greater efficiency and effectiveness.
Optimization principles, practices, and methods can be used to evaluate different stages of the remediation process, and the goals may be different for each stage. The key components of the optimization process and the remedial phases at which optimization can be applied are shown in Figure 2‑1. USEPA’s independent optimization review program focuses on protectiveness, effectiveness, cost-efficiency, technical improvement, progress toward site completion, and environmental footprint reduction. An optimization review considers the goals of the remedy, available site data, the CSM, remedy performance and trends, and the exit strategy (e.g., transition to passive remediation, or site closure).
2.1.3 Current Approaches for P&T Optimization
P&T optimization has been an ongoing effort since the 1990s through the United States Army Corps of Engineers (USACE), DOD, and USEPA. USEPA has been conducting optimization reviews and technical support for certain projects since 1997, especially for P&T remedies focusing on process optimization and long-term monitoring for achieving remedy objectives. An agency-wide directive was issued in 2012 that expanded and formalized the optimization practices from site assessment to site completion for all remedies under the Superfund program ( USEPA 2012[ENEAAYBS] USEPA. 2012. “National Strategy to Expand Superfund Optimization Practices from Site Assessment to Site Completion.” U.S. Environmental Protection Agency, Office of Solid Waste and Emergency Response. https://nepis.epa.gov/Exe/ZyPURL.cgi?Dockey=P100GI85.txt. ). The purpose of this directive was the integration of new practices to promote increased efficiency and effectiveness at all stages of the CERCLA process at all Superfund sites.
In addition to efforts by the USEPA, state/federal agencies and other entities have implemented their own optimization programs in the last several decades to leverage the benefits of periodic optimization of remediation systems for cost avoidance and to improve remedy effectiveness. Although periodic optimization of remedy systems is not a regulatory requirement, remedy optimization—conducted in conjunction with periodic evaluation of remedial system performance—has provided significant benefits to decision-makers, regulators, and stakeholders. Approaches developed to provide high-level optimization assessment include the ITRC documents Remedial Process Optimization (RPO-1 to -6) and the RSEs ( USEPA 2013[4DYZQGUT] USEPA. 2013. “Remediation Optimization: Definition, Scope and Approach.” U.S. Environmental Protection Agency. https://clu-in.org/Optimization/pdfs/OptimizationPrimer_final_June2013.pdf.ITRC 2004[JKA62F2T] ITRC. 2004. “Remediation Process Optimization: Identifying Opportunities for Enhanced and More Efficient Site Remediation.” Washington, D.C.: Interstate Technology & Regulatory Council, Remediation Process Optimization Team. https://itrcweb.org/teams/projects/remediation-process-optimization. ). These optimization assessment approaches recognize the potential inefficiencies associated with the traditional study-design-build linear approach and instead aim to manage uncertainties that result in remedy performance limitations through a systematic evaluation of remedy objectives and continuous improvement and optimization of remedy technologies as typically involved in adaptive management. Briefly, these approaches involve a remedy review by an independent team to assess progress toward achieving remedy objectives and to provide recommendations for system optimization. The original RSEs focused only on engineered remedies, but optimization today has a much broader scope.
Several of these independent optimization efforts were later unified under a strategy developed by USEPA to expand and formalize optimization practices for the Superfund (CERCLA) program ( USEPA 2012[ENEAAYBS] USEPA. 2012. “National Strategy to Expand Superfund Optimization Practices from Site Assessment to Site Completion.” U.S. Environmental Protection Agency, Office of Solid Waste and Emergency Response. https://nepis.epa.gov/Exe/ZyPURL.cgi?Dockey=P100GI85.txt. ). This strategy emphasizes the importance of applying optimization concepts to support decision-making throughout all phases of the Superfund project lifetime. Application of this broader optimization approach has been shown to achieve significant cost savings at many sites while meeting the remedy objectives ( USEPA 2013[4DYZQGUT] USEPA. 2013. “Remediation Optimization: Definition, Scope and Approach.” U.S. Environmental Protection Agency. https://clu-in.org/Optimization/pdfs/OptimizationPrimer_final_June2013.pdf. ). These strategies provide clear guidance to effectively identify optimization needs due to changes in conditions such as a revised regulatory framework, identification of a new treatment technology or a new contaminant at a site, or changes in land use near the system. Often, these approaches include a dynamic framework where updates to the CSM guide mass removal and containment adjustments.
Additional details of existing optimization programs and approaches are provided in Appendix D, Existing Optimization Programs.
2.2 Performance-Based Pump and Treat Optimization
Remedy performance evaluations for P&T operations are often required by some regulatory agencies, and recommended by USEPA, to demonstrate compliance, track progress toward achieving cleanup goals, and support decision-making for remedy modifications and site completion (or transition to other remedies). Three main components of performance assessments are (1) evaluation of the aboveground treatment system performance, including the conveyance piping and equipment, the treatment system, and the discharge process; (2) evaluation of the subsurface performance including plume capture and containment, recovery performance for plume(s) (and source when relevant), and performance of the extraction-well and injection-well networks; and (3) monitoring (belowground and aboveground), reporting, project management, contracting, and sustainability. Additional details and tools for conducting an optimization review of a P&T system during different steps of site remediation are provided in Sections 3 and 4. A performance-based optimization approach relies on frequent updates to the CSM based on the data and information collected during operations to inform further adjustments to the system ( USEPA 2011[B5FVZMT4] USEPA. 2011. “Environmental Cleanup Best Management Practices: Effective Use of the Project Life Cycle Conceptual Site Model.” Office of Solid Waste and Emergency Response. EPA 542-F-11-011. https://www.epa.gov/sites/default/files/2015-04/documents/csm-life-cycle-fact-sheet-final.pdf. ). This approach considers the current or changing conditions associated with the nature of the source and/or the contaminants of concern (COC) plume (e.g., additional sources, additional mass of contaminant, changing concentration spatial distributions, contaminant degradation, etc.), as well as aspects controlling contaminant transport (e.g., aquifer hydraulic conditions) and remedy performance (e.g., well design and pumping performance).
A similar structured approach for P&T remedy performance evaluations was developed. It consists of several primary evaluations and supports decision outcomes such as the need for optimizing the P&T system ( USEPA 1988[5EUSVMWV] USEPA. 1988. “Guidance for Conducting Remedial Investigations and Feasibility Studies Under CERCLA.” U.S. Environmental Protection Agency. ). These approaches, along with advancements in the use of predictive fate and transport evaluations and integration of optimization methods, allow an adaptive management strategy to be implemented for well-network design and management for P&T operations. A similar phased or integrated approach has been highlighted in several USEPA guidance documents to prevent over-designing initial P&T systems to account for uncertainty in site characterization and instead recommend implementation that is governed by a set of system expansion requirements ( USEPA 2013[4DYZQGUT] USEPA. 2013. “Remediation Optimization: Definition, Scope and Approach.” U.S. Environmental Protection Agency. https://clu-in.org/Optimization/pdfs/OptimizationPrimer_final_June2013.pdf. ). An adaptive strategy can facilitate dynamic changes in pumping strategies; for example, an initial capture bulk treatment approach can be fine-tuned or modified over time based on system characteristics (e.g., related to changes in plume size, distribution, remedy efficiencies, etc.) and a refined CSM.
2.2.1 Pump and Treat Performance Evaluations
More detailed information on P&T remedy performance evaluations is provided in Section 3, but this section provides a high-level summary of the process. In general, hydraulic plume containment evaluations for each containment area (there may be multiple separate areas that need to be contained at a given site) involve monitoring hydraulic heads and gradients, analytical calculations and/or numerical modeling of capture zones relative to compliance boundaries, monitoring groundwater quality to establish and evaluate concentration trends over time, particle tracking using numerical fate and transport modeling to establish or confirm a predicted flow system, and potentially, implementation of tracer tests to confirm capture. Collectively, these analyses and data can provide the necessary lines of evidence to ensure capture and inform changing conditions to update the CSM and assess the pore volume flushes needed and the time it will take to provide these flushes, given the P&T configuration, to achieve cleanup goals. For example, an increasing concentration trend around the capture zone for a source area may indicate additional fluxes into the groundwater requiring more aggressive capture in the vicinity of the zone ( USEPA 2013[4DYZQGUT] USEPA. 2013. “Remediation Optimization: Definition, Scope and Approach.” U.S. Environmental Protection Agency. https://clu-in.org/Optimization/pdfs/OptimizationPrimer_final_June2013.pdf. ). Moreover, increasing contaminant trends downgradient of a pumping or capture zone are clear indications that full capture is not occurring. Reevaluation of the capture zone and changes in the direction of groundwater flow may indicate the need for additional extraction well(s).
Treatment process monitoring refers to measurements of influent flow rate to the treatment plant and concentrations in treatment plant influent and effluent and at intermediate points in the treatment process (see Section 3). One of the primary criteria of evaluation is the verification that discharge standards are being achieved. Approaches such as comparing design parameters to actual parameters for the treatment system, such as contaminant mass loading or contaminant mass removal rates, can further inform the treatment system evaluations. The process monitoring data can be used to determine whether processes are working according to design, require maintenance, need to be replaced with a different process, or are no longer needed.
The goals of P&T implementation for the purposes of aquifer restoration or plume reduction typically include reducing contaminant concentrations to Clean Water Act Maximum Contaminant Levels (MCLs) or drinking water standards, maximizing contaminant mass removal, minimizing cleanup time, and minimizing cost. Effectiveness of a subsurface remedy depends upon the accuracy and completeness of the CSM and, therefore, often requires a high degree of performance monitoring to address site uncertainties that may significantly impact system performance. The frequency of monitoring is based on the rate of change of hydraulics, contaminant trends, and geochemical changes in the subsurface and proximity to receptors, which are important aspects of the CSM. The resulting information is ideally used for frequent improvements to the CSM. The CSM is developed and updated based on USEPA’s Project Life Cycle CSM shown in Figure 2‑1 ( Clu-In 2016[N7J8G5Q3] Clu-In. 2016. “Key Optimization Components: Conceptual Site Model.” Contaminated Site Clean-Up Information. 2016. https://clu-in.org/optimization/components_csm.cfm. ). These performance analyses involve frequently updated plume maps, cross-sections, and/or 3DVA based on monitored groundwater quality data and hydraulic data, potentiometric head surfaces, measured groundwater flow directions and gradients, and data trend plots ( USEPA 2011[B5FVZMT4] USEPA. 2011. “Environmental Cleanup Best Management Practices: Effective Use of the Project Life Cycle Conceptual Site Model.” Office of Solid Waste and Emergency Response. EPA 542-F-11-011. https://www.epa.gov/sites/default/files/2015-04/documents/csm-life-cycle-fact-sheet-final.pdf. ). Updating the understanding of geology and stratigraphy (e.g., low permeability lenses, preferential flow pathways) as well as the contaminant distribution aspects of the CSM is an essential precursor activity for assessing the performance of the current remedy design and supporting further decisions on potential optimization actions. The typical parameters to measure include the concentrations of contaminants, chemicals that could affect the treatment system, and chemicals that may indicate the presence of other processes of interest, such as natural attenuation. Actual and projected concentration trends from individual wells may be used to evaluate the overall cleanup performance, assess cleanup time frames, and identify issues such as tailing or rebound. Furthermore, with plume maps, cross-sections, and 3DVA products, trends in contaminant distribution in groundwater also provide an assessment of remedy and restoration progress.
Another component of P&T evaluations is the evaluation of extraction well performance. Monitoring of the contaminant concentrations and groundwater levels, compared against predicted performance, along with evaluating the pumping effectiveness of the extraction wells themselves, help determine whether the extraction-well network configuration is continuing to provide the most effective and efficient recovery. This evaluation, most often conducted individually for each well, along with the other components of the performance evaluation, should indicate whether adjustments to the pumping rates, well cleaning and/or rehabilitation, and/or installation of new wells is needed to maintain or increase the recovery efficiency. Similarly, injection well capacity and locations are an important component of performance evaluations at sites where injection wells are located upgradient of extraction wells and are used for returning the treated P&T effluent back to the aquifer. In many systems, these wells are used to hydraulically manage and maintain flow conditions as well as support flushing of contaminants through the subsurface.
Information collected can be used to evaluate essential aspects of the P&T system, including a range of pore volume flushes estimated to achieve the cleanup goals, issues related to stagnation zones or plume containment, progress toward remedial goals through analyses such as comparing mass removal and residual mass trends, and effective coverage of the monitoring-well network for contamination and hydraulic head monitoring. The number of monitoring wells at which samples are collected (in addition to the extraction wells) and the sampling frequency depend on site-specific conditions and cost-benefit tradeoffs. In general, greater sampling density and frequency are likely to improve the CSM, particularly during the earlier period of operation, and allow more adaptive and effective P&T remediation ( USEPA 2013[4DYZQGUT] USEPA. 2013. “Remediation Optimization: Definition, Scope and Approach.” U.S. Environmental Protection Agency. https://clu-in.org/Optimization/pdfs/OptimizationPrimer_final_June2013.pdf. ).
Detailed information on the performance evaluations for P&T systems can be found in Section 3.
2.2.2 Elements of Performance-Based Optimization
A performance-based P&T optimization approach is built on an adaptive management framework where routine performance evaluations provide the basis for identifying and/or tracking changing site conditions, updating the CSM, and identifying adjustments to the remedy. This approach has the potential to expedite cleanup, incrementally reduce uncertainties with targeted optimization activities, support effective and efficient remedy performance, and optimize costs and cleanup time frame. It can result in increases in the pore volume flushes needed and/or reductions in the time it will take to provide these flushes to achieve cleanup goals.
Adaptive Site Management (ASM) Adaptive site management (ASM) is a systematic and iterative site management approach recognized for the potential benefits of improving and expediting cleanup of large and/or complex hazardous waste sites. The USEPA Superfund program defines ASM as a formal and systematic project or site management approach centered on rigorous site planning and a firm understanding of site conditions and uncertainties. It is defined as a technique, rooted in the sound use of science and technology, that encourages routine reevaluation and management prioritization of site activities to account for new information and changing site conditions. The goal of the approach is to create a framework of structured and continuous planning, implementation, and assessment processes that targets management and resource decisions to incrementally reduce site uncertainties and support effective and efficient site progress that achieves the required outcome(s) while optimizing costs, cleanup time frame, and performance. ASM is expanded upon in Common Concepts (Appendix A). ( ITRC 2017[GMA6VGKV] ITRC. 2017. “Remediation Management of Complex Sites.” Washington D.C.: Interstate Technology & Regulatory Council, Remediation Management of Complex Sites Team. https://rmcs-1.itrcweb.org/.Demirkanli and Freedman 2021[TBSE8KK9] Demirkanli, D.I., and V.L. Freedman. 2021. Adaptive Site Management Strategies for the Hanford Central Plateau Groundwater. Richland, WA: PNNL-32055. ) |
As shown in Figure 2‑4, following an initial optimization of a P&T remedy, performance assessments conducted at the site can help update the CSM periodically and will identify whether the remedy performance is evolving as predicted and whether the remedy is likely to achieve RAOs. CSM updates may initiate further remedy optimization actions. These optimization elements include the following:
- optimization of the operation, maintenance, and monitoring effectiveness of the treatment system for the extracted groundwater
- adjustments to the network of extraction wells and/or trenches and operations for hydraulic containment, contaminant removal, and/or aquifer restoration
- optimization of the treated water injection well and/or discharge network
- optimization of the groundwater and treatment monitoring systems
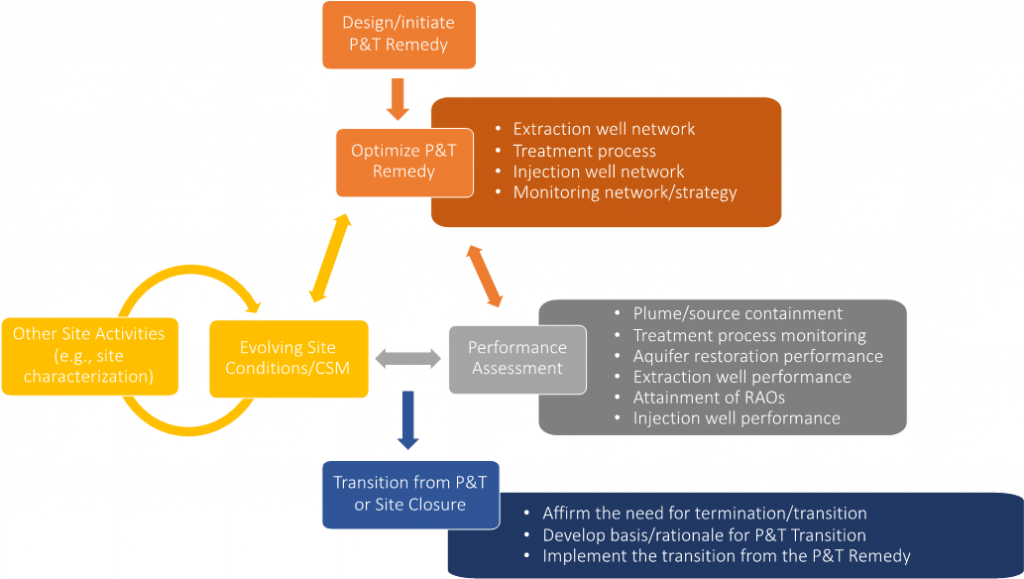
These represent the primary technical elements of optimization specific to the P&T technology that are needed to support an overall remedy optimization effort. One of the most critical elements for P&T performance optimization in relation to plume remediation efficiency and effectiveness is adapting the well-network approach (well locations, well screen depth/well construction, and pumping rates) to evolving plume conditions as the remedy progresses. For more information on the performance-based optimization approaches, see Section 4. If transition from P&T to a different remediation approach is being considered due to nonattainment of RAOs, other remediation technologies should be evaluated prior to transitioning to another approach to determine whether they can reasonably attain RAOs.
2.2.3 Potential Optimization Impacts
Typically, optimization of groundwater pump and treatment systems may result in cost savings and/or reduction in remedy lifetime. When implemented dynamically and based on site-specific remedy performance assessments, optimization efforts are expected to incrementally reduce the O&M costs and accelerate the cleanup time frame (Figure 2‑5). Optimization can also be performed for a variety of reasons that do not necessarily result in immediate cost savings, such as risk reduction due to plume migration or exposure, the need to treat emerging contaminants, and sustainable and resilient remediation considerations.
Figure 2‑5 depicts effort and cost over time for an idealized P&T effort at a contaminated site. In the initial stages of a site response, much of the effort is necessarily focused on characterization, risk determination, remedy evaluation and selection, and source control and remediation. As the project matures and the P&T remedy is selected for the long-term active treatment, resources are increasingly spent on monitoring and P&T system O&M. Manual labor costs related to O&M, project management, and reporting are typically a major cost driver. Some systems require multiple people to operate and maintain them. As indicated by the dashed line at “site closure or transition from P&T,” technical and remedy performance uncertainties may impact the accuracy of the estimates for total long-term costs. However, as seen in Figure 2‑5, an incrementally applied, performance-based optimization throughout the lifetime of the remedy has the potential to reduce costs as well as the active cleanup time frame. The costs of monitoring and treatment system O&M can be reduced over time, eventually allowing the transition of the remedy to MNA or site closure. Optimization reviews can result in substantial cost avoidance and savings and enable reductions in time to achieve site completion, although such results depend on site-specific conditions.
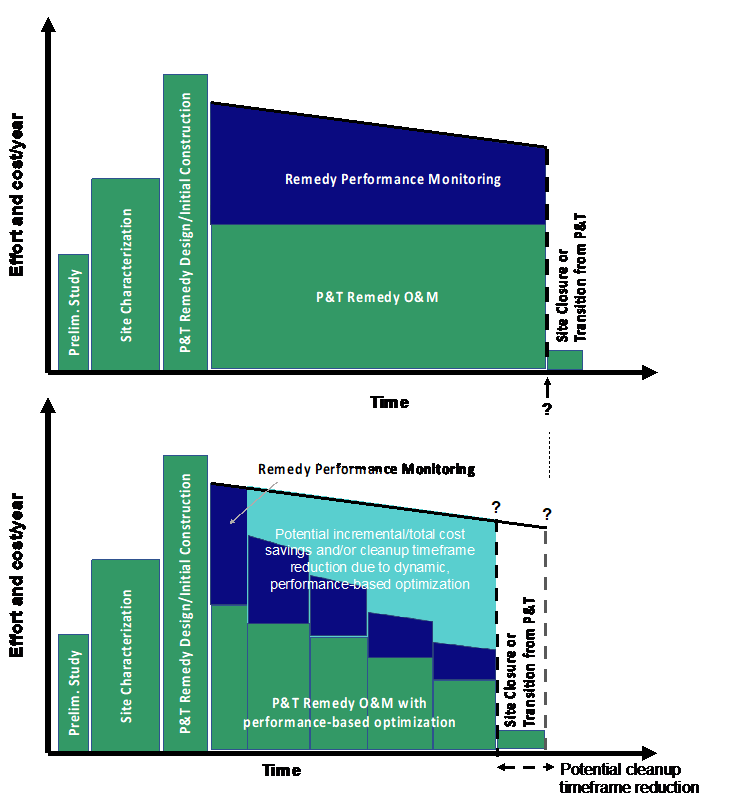
Adapted from: ITRC Remediation Process Optimization: Identifying Opportunities for Enhanced and More Efficient Site Remediation ( ITRC 2004[JKA62F2T] ITRC. 2004. “Remediation Process Optimization: Identifying Opportunities for Enhanced and More Efficient Site Remediation.” Washington, D.C.: Interstate Technology & Regulatory Council, Remediation Process Optimization Team. https://itrcweb.org/teams/projects/remediation-process-optimization. ).
Optimization has a greater ability to influence life cycle costs if performed more dynamically as the new information is integrated into CSM during remedy performance assessments or evaluations (Figure 2‑6). Early incorporation of independent optimization reviews or direct application of optimization lessons learned during the remedy selection, design, and procurement phases of work can also be effective. As shown in Figure 2‑6, as operational data are obtained and new remediation technologies become available, there may be opportunities to increase the ability to influence remediation costs through CSM refinement, remedy modification, and streamlining of remedy progress monitoring.
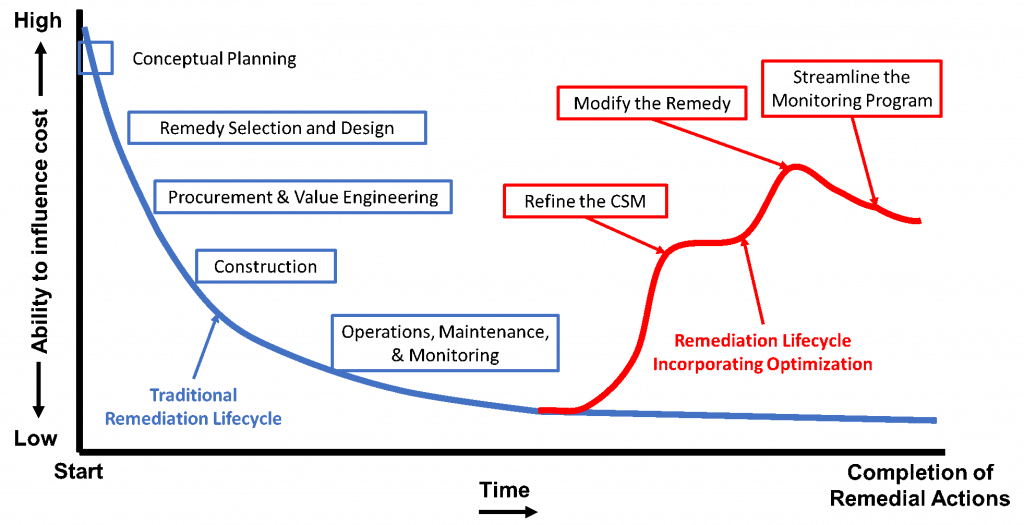
Adapted from: ITRC Remediation Process Optimization: Identifying Opportunities for Enhanced and More Efficient Site Remediation ( ITRC 2004[JKA62F2T] ITRC. 2004. “Remediation Process Optimization: Identifying Opportunities for Enhanced and More Efficient Site Remediation.” Washington, D.C.: Interstate Technology & Regulatory Council, Remediation Process Optimization Team. https://itrcweb.org/teams/projects/remediation-process-optimization. ).
2.3 Interactive Tool for Assessing Whether Optimization Might Be Beneficial for Your Site
An interactive tool for assessing whether performance of an optimization review may benefit a site with a P&T-based remedy is provided in Appendix C.
The tool is based on the USEPA remedial process optimization (RPO) checklist included in Appendix C. It was constructed from a series of questions used to assess the state of knowledge about site conditions and how the site could benefit from an optimization effort. They start with general questions about the accuracy of the site’s CSM and progress toward RAOs and then to more detailed questions about the use of supervisory control and data acquisition (SCADA) systems, energy assessments, and stakeholder involvement.
The potential to benefit from optimization of the remediation system is determined from the responses to the questions. The responses to the individual questions are qualitative in nature and categorize the potential for optimization as high, medium, or low. The results are then compiled to produce an overall assessment of the potential to benefit from an optimization review. Links to different sections in this document are provided for the questions and offer the user of the tool a way to learn more about each of the topics and thus provide more informed responses to the questions.
Key Takeaways
|