3 Pump and Treat Performance Evaluation
P&T systems are typically designed and implemented to protect human and ecological receptors from the impact of COCs migrating in the groundwater. They accomplish this and the P&T system’s RAOs through source control, source remediation, hydraulic and chemical plume containment, and/or plume reduction by means of groundwater extraction and treatment to achieve the regulatory discharge limits or RAOs. Like the CERCLA five-year reviews, the performance of P&T systems should be evaluated periodically, preferably on a set schedule, to determine their effectiveness and efficiency in protecting the receptors and their progress toward achieving the RAOs. In addition, the P&T system should be evaluated to assess equipment performance, including its useful life, as well as energy efficiency and resilience to climate change. Performance inspections and evaluations should also be performed after major events, such as fires or storm damage, and after a milestone or benchmark is achieved.
State Survey Question 5 How are states currently evaluating P&T systems?
See Appendix E for full state survey results. |
Below is the Optimization Life Cycle Navigation Diagram. Performance evaluation is the second step.
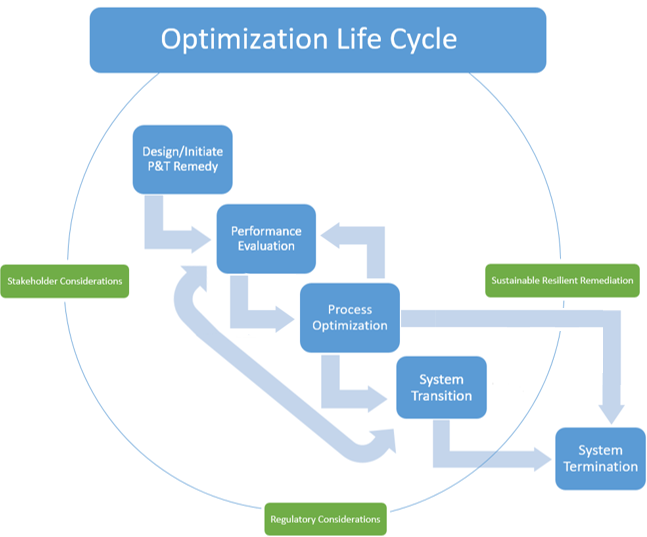
Optimization Life Cycle Navigation Diagram.
E. Madden, ITRC. Used with permission.
The P&T performance evaluation process of existing P&T systems is discussed in this section; it is similar to the Optimization Review in the USEPA National Optimization Program. The information obtained as part of this evaluation is used in the P&T optimization process, discussed in Section 4, and in evaluating strategies in Section 5; it is similar to the Optimization Review Recommendations in the USEPA National Optimization Program.
3.1 System Components, System Evaluation Process, and Drivers of Performance Evaluation
This subsection presents the components of the P&T system that undergo performance-based optimization and discusses the steps and drivers of the performance evaluation as the initial element in the overall assessment of the system.
3.1.1 Components of a Typical Pump and Treat System
A P&T system typically consists of the following major physical components as discussed in more detail in Section 2.1:
- aquifer interception
- conveyance system
- contaminant control, alteration, reduction, or destruction
- discharge system
- energy/operation
- monitoring system
A performance-based optimized P&T system is one that undergoes periodic evaluations of performance followed by adjustments of its physical elements (e.g., optimization) with the goal of advancing the remedial action to the RAOs. This approach protects human and ecological receptors in an efficient and cost-effective manner.
3.1.2 Performance Evaluation Process
Common steps included in the P&T system performance evaluation process are identified below. The inclusion and order of executing these steps are project specific. P&T performance evaluation is an iterative process; therefore, some steps may be included multiple times as data become available.
- Develop a performance evaluation team.
- Determine whether the system is functioning as designed (are RAOs projected to be met in a reasonable time frame?). Assess the effectiveness and efficiency with which objectives are met (e.g., is there sufficient hydraulic and chemical capture of the plume, are the discharge limits being met, is the treatment equipment nearing the end of its useful life?).
State Survey Question 12 Does your state have regulations for any of the following concepts that apply to P&T systems?
See Appendix E for full state survey results. |
- Establish whether the project objectives require updating (e.g., are points of compliance still applicable (see ITRC 2017[GMA6VGKV] ITRC. 2017. “Remediation Management of Complex Sites.” Washington D.C.: Interstate Technology & Regulatory Council, Remediation Management of Complex Sites Team. https://rmcs-1.itrcweb.org/. ), are the receptors protected, have the receptors or the discharge limits for the treated water changed since the time of the design/implementation?).
- Assess the availability of the data required to conduct the performance evaluation (e.g., is the CSM sufficient to analyze the system? Update the CSM as necessary. Are sufficient operational, system hydraulics, and hydrogeologic data available to complete an objectives-driven evaluation?).
- Evaluate the adequacy of the monitoring system (e.g., is the current monitoring system capable of determining the degree of plume containment or demonstrating compliance?).
- Obtain additional data (e.g., performing specific capacity testing of the extraction wells after assessing operational trends such as decreasing flow or increasing drawdown levels in one or more extraction).
- Perform additional focused evaluation studies as needed (e.g., supplemental characterization activities, plume modeling, and trend analysis).
- Conduct the preliminary investigation of the cost-effectiveness of the P&T operation (e.g., identifying the major cost item of the P&T operation). This step can be further developed as part of the optimization process, discussed in Section 4.
- Conduct the preliminary assessment to determine whether P&T is still the appropriate technology for the site (e.g., can the project objectives still be achieved using the current P&T approach, is P&T the most cost efficient compared to other technologies?). This is further discussed in Sections 4 and 5 as optimization and transition strategies.
State Survey Question 13 Does your state have guidance materials for any of the following concepts that apply to P&T systems?
See Appendix E for full state survey results. |
- Evaluate the system’s ability to be sustainable and resilient to changing environmental conditions (see Section 6).
- Prepare the performance evaluation report.
The performance evaluation process should use multiple lines of evidence. The frequency of the evaluation events is project specific and should be established by the project team. The results of the evaluation will allow the project team to determine whether proceeding to system optimization is warranted and, if so, will directly inform the establishment of the goals of the optimization process. The optimization process is discussed in Section 4.
3.1.3 Performance Evaluation Drivers
This subsection discusses the two types of drivers (internal and external) that may trigger the need for evaluating the performance of the P&T system. Common evaluation drivers are identified; however, additional project-specific drivers may be developed by the project team.
3.1.3.1 Internal Drivers
Internal system drivers are directly related to the current system performance. They include factors such as the following:
- Collection of new information on the site hydrogeology or contaminant fate and transport that substantially change the understanding of the subsurface conditions and may require reevaluation of the system design and an update to the CSM.
- Observation of the loss of plume capture, loss of capacity of the extraction wells, bypass of the plume between wells, or inability to achieve the required treatment levels. The reason for the performance deterioration should be investigated and determined.
- Unexpected outcomes including, but not limited to, redirection of the plume into a previously unaffected area, dewatering of aquifers or wetlands, etc. The underlying reason should be evaluated and identified.
- Observation of the loss of efficiency of the system, such as slower than expected rate of remediation, eroding treatment system performance, or decreasing cost-effectiveness. The reason for the inefficiency should be investigated.
3.1.3.2 External Drivers
The external drivers are factors that do not directly impact the current system performance; nevertheless, they may require that the system be reevaluated. They include the following:
- Reduction in the operating budget of the site. The operation of the system may need to be reassessed to identify methods to continue achieving the project objectives with the reduced budget.
- New external hydraulic stresses such as new irrigation and other water supply changes.
- Interaction with stakeholders. Stakeholders may generate new requirements that require reevaluation of the project objectives. Examples include plans to develop the site or adjacent properties, changes in ownership, or changes to the makeup of the surrounding community. This is further discussed in Section 8.
- Regulation changes may require that the system operation be adjusted. Examples include the identification of new contaminants of emerging concern (CECs) and the requirement to treat them, new monitoring requirements, changes to the discharge limits, adoption of new methods for managing the risk to receptors, or new land use regulations. This is further discussed in Sections 4.2.2, 4.2.3, and 7.3.
- Environmental concerns or benefits, such as the need to implement the principles of green remediation and increase climate resiliency (see Section 6).
3.2 Identifying Data Gaps and Updating the Conceptual Site Model
During the performance evaluation process, it may become evident that a P&T system lacks effectiveness due to a misunderstanding or incomplete understanding of site conditions. The existing CSM must be reviewed and gaps in knowledge identified (e.g., data gaps), as necessary, so the CSM, and consequently the P&T system, can be modified and optimized to achieve remediation goals. A focused evaluation of the existing data identifies data gaps that affect the evaluation of the operational efficacy and constraints within the system equipment, instrumentation, and infrastructure (e.g., pumps, review of SCADA parameters and records, conveyance, tanks, or treatment unit). This may require only a reinterpretation of existing data, or it may warrant the collection of additional site characterization data and an update of the CSM.
The components of a CSM encompass all aspects of a site and its condition including hydrogeology, contaminant sources and distribution, treatment system performance, and receptors; data gaps may exist in some or all of these components. Inadequate characterization of subsurface heterogeneity is commonly the principal source for data gaps at most sites. Many of the available tools to address the characterization of subsurface heterogeneity are described in ITRC’s Advanced Site Characterization Tools guidance document ( ITRC 2019[ZMM54HEL] ITRC. 2019. “Implementing Advanced Site Characterization Tools.” Washington, D.C.: Interstate Technology & Regulatory Council, Implementing Advanced Site Characterization Tools Team. https://asct-1.itrcweb.org/. ). Once data gaps are identified and addressed to the extent feasible, the CSM should be updated in accordance with previously published guidance documents ( ITRC 2012[JVPK234H] ITRC. 2012. “Incremental Sampling Methodology.” Washington, D.C.: Interstate Technology & Regulatory Council, Incremental Sampling Methodology Team. https://www.itrcweb.org/ism-1/. , ITRC 2018[QJNTKDVM] ITRC. 2018. “TPH Risk Evaluation at Petroleum-Contaminated Sites.” Washington, D.C.: Interstate Technology & Regulatory Council, TPH Risk Evaluation Team. https://tphrisk-1.itrcweb.org/. , USEPA 2011[B5FVZMT4] USEPA. 2011. “Environmental Cleanup Best Management Practices: Effective Use of the Project Life Cycle Conceptual Site Model.” Office of Solid Waste and Emergency Response. EPA 542-F-11-011. https://www.epa.gov/sites/default/files/2015-04/documents/csm-life-cycle-fact-sheet-final.pdf. ).
Several case studies (Appendix B) highlight concerns related to data or operational uncertainties that create data gaps that affect meeting the RAOs. Several of these case studies include an effort to update the CSM using the existing data, collection of supplemental data to address data gaps and modifications to the monitoring programs. Three examples are listed below:
- Saco Defense, Maine—Use of a hydraulic barrier extraction well system is adequate to contain the bulk of plume, but the source zone depletion rate is extremely slow. Evaluation relied on existing data and understanding of matrix-diffusion effects within the stagnant source zone. Two scenarios were modeled: one was enhanced anaerobic dechlorination (EAD), and the other was a source zone pumping well. Two versions of RemChlor were used. The 2018 version did not take back diffusion into account and prompted the design of the EAD. The 2020 version included the effects of back diffusion. Once back diffusion was included, the models indicated that neither enhancement would significantly reduce risk or time and would only increase cost. The barrier system continues operating, and MNA downgradient of the pumping wells reduces risk to off-site receptors sufficiently.
- Benfield Industries, North Carolina—The aquifer-plume intercept is not effectively connected hydraulically to the source zone (it pulls in an inordinate amount of “clean groundwater” from underlying saprolite instead of from primary source–contributing alluvial materials). Following a staged approach designed to collect additional data to evaluate remedial alternatives, data gaps associated with source conditions provided a clearer path to select ISCO as the preferred remedy for targeting this source alluvium.
- DOD Installation 2—This site has multiple aquifer-unit extraction systems (shallow and deep) separated by a discontinuous aquitard. Operations were complicated by overlapping hydraulic functions (e.g., hydraulic head and associated gradients) with a recharge gallery, operational issues (electrical and biofouling) in the shallow unit wells, and a capture zone that appeared to be influenced (shifting laterally off the installation boundary). Existing data were supplemented with additional data to address these observations and concerns and to support model calibration, optimization of treatment systems, well rehabilitation, and possible interception of contaminated shallow groundwater before it migrated through discontinuities in the aquitard and impacted groundwater in the deeper unit.
3.3 Performance Assessment
The performance of a P&T system should be assessed regularly to ensure that it continues to achieve project objectives or to establish the need for adjustments to operations or objectives. Following the update of the CSM, if necessary, as discussed in Section 3.2, a performance assessment begins with a baseline comparison. A baseline comparison involves revisiting the project objectives and analyzing the existing data. Revisiting the objectives involves focusing on the existing objectives, revising the project objectives to the new reality, or modifying the objectives to support the next generation of remedy/corrective measure. In all cases, a shared understanding of the stakeholders’ concerns and goals is key. Existing data (e.g., operations, monitoring, equipment downtime/failures) and the current CSM provide the baseline for further analysis. They allow the practitioner to identify the most important data needs and reduce the cost of the subsequent investigation.
USACE (see link in Appendix D) has an instruction guide and a checklist for remedy system evaluation that are helpful when assessing the performance of P&T systems.
P&T system performance evaluation relies on the available data (e.g., extraction rates, water elevations, hydraulic gradients, and contaminant concentrations, etc.). Collecting sufficient, reliable, and usable data is very important for system performance evaluation. O&M of a P&T system should include maintaining a well-documented O&M plan and sampling and analysis plan, which should clearly state which data are going to be collected, how the data will be collected and analyzed, data collection frequency, and data review procedures. These plans should be updated periodically to build on lessons learned as the site matures and as site conditions change. A performance evaluation plan is highly recommended to provide an overarching methodology and framework for conducting the performance evaluation. In this section, assessment of the main components of the P&T system is presented: extraction and monitoring, injection and recirculation, modeling, network considerations, treatment, and conveyance. The use of modeling is discussed as an important method to assess the overall performance of the P&T remedy.
3.3.1 Extraction and Monitoring Evaluation
The extraction and monitoring system evaluation focuses on assessing the effectiveness and efficiency of extraction wells with respect to plume containment and/or contaminant mass removal and the sufficiency of monitoring wells in defining plume boundaries and indicating effectiveness of the remedy.
3.3.1.1 Evaluation of Extraction Effectiveness
Performance of extraction can be evaluated by examining multiple lines of evidence including, but not limited to, the data on the following aspects of the system: 1) extraction well performance, 2) plume containment and stability, 3) NAPL removal, and 4) groundwater quality restoration.
3.3.1.1.1 Evaluation of Extraction Well Performance
Extraction effectiveness of a P&T system depends on the placement and performance of extraction wells. Performance of extraction wells should be monitored routinely (e.g., monthly or quarterly) to ensure that extraction wells operate at designed settings so that deterioration of wells can be detected and corrected in a timely manner. Extraction-well performance is affected by the operation of the well pump, motor, associated piping, and well screen and filter pack. Pumping rates from extraction wells directly reflect the operating condition of the wells. Each extraction well should have a designated or targeted pumping rate and/or up-time set from the remedial design. The actual pumping rate from each extraction well should be monitored and compared with design values. If the actual pumping rates are different from design values, the extraction well and conveyance system should be investigated. Evaluation of extraction-well performance should include monitoring of water levels (with and without pumping) to assess whether the erosion of the extraction rate could be caused by the decrease of ambient potentiometric heads and monitoring the trend of specific capacity (pumping rates over drawdown levels) (see Appendix A. Common Concepts). Continued drop of well capacity indicates performance degradation or potentially dewatering of the aquifer, and well rehabilitation or other corrective measures such as modification or replacement/abandonment need to be considered as discussed in Section 4.3.1.6. More details on the evaluation of extraction-well performance is provided in Section 3.3.4.
3.3.1.1.2 Evaluating Plume Containment and Plume Stability
Hydraulic and chemical containment (capture) of the plume is one of the main goals at most contaminated groundwater remediation sites. Hydraulic containment or capture controls movement of contaminated groundwater to prevent continued plume expansion and further migration, and it is an important method of maintaining plume stability. Plume containment or capture can be evaluated through monitoring, analysis, and modeling. The evaluation tools include but are not limited to interpretation of groundwater elevation data (e.g., inward hydraulic gradient through boundaries like a slurry wall indicting containment), monitoring concentration trends, capture analysis, tracer test analysis, and particle tracking in conjunction with modeling (see Appendix A) ( USEPA 2002[DQBFZY38] USEPA. 2002. “Elements for Effective Management of Operating Pump and Treat Systems.” U.S. Environmental Protection Agency, Office of Solid Waste and Emergency Response. https://www.epa.gov/sites/default/files/2015-04/documents/factsheet_for_pt.pdf.USEPA 2008[7ILB4T4V] USEPA. 2008. A Systematic Approach for Evaluation of Capture Zones at Pump and Treat Systems. Ada, OK: U.S. Environmental Protection Agency, National Risk Management Research Laboratory. https://cfpub.epa.gov/si/si_public_record_report.cfm?Lab=NRMRL&dirEntryId=187788. ).
Groundwater elevation and contaminant concentrations are two important measurements in plume containment assessment. Groundwater elevation data are often used to create potentiometric surface maps from which groundwater flowlines and a capture zone can be interpreted ( USEPA 2002[DQBFZY38] USEPA. 2002. “Elements for Effective Management of Operating Pump and Treat Systems.” U.S. Environmental Protection Agency, Office of Solid Waste and Emergency Response. https://www.epa.gov/sites/default/files/2015-04/documents/factsheet_for_pt.pdf. ); however, insufficient elevation data or a lack of understanding of aquifer heterogeneity and anisotropy may limit the accuracy of interpretation. In addition to water elevation from each well, paired groundwater elevation data on both sides of a boundary can be used to evaluate hydraulic containment through boundaries like a slurry wall; an inward hydraulic gradient normally indicates efficient containment. Compared to interpretation from a potentiometric map, monitoring contaminant concentration trends at monitoring wells, especially at sentinel monitoring wells (monitoring wells established between a plume front and a receptor) and wells near plume boundaries, is more a more straightforward way to evaluate whether the plume has been efficiently contained and become stable (i.e., no further expansion or migration). For some P&T systems, COC concentration trends are not consistent at all wells within or downgradient of the plume. Some trends show increases, some show decreases, and some remain stable. Consistently decreasing or stable concentration trends at sentinel monitoring wells (i.e., no change between two or more monitoring periods) may suggest that plume expansion is sufficiently contained. On the other hand, an increasing concentration trend at a downgradient well may indicate inadequate capture, at least locally if no additional source is apparent. (See Appendix A for a comprehensive review of the types of trend analysis that can be applied to evaluate P&T performance.)
Compared to interpretation of potentiometric surface maps and concentration trend monitoring for individual wells, comprehensive capture analysis can be more complicated and may require more extensive data interpretation and evaluation (potentiometric surface map, water level pairs and concentration trends, etc.), calculation (estimated flow, capture zone width), and modeling (predicted capture). USEPA has a guidance document for a systematic approach to capture zone analysis of P&T systems ( USEPA 2008[7ILB4T4V] USEPA. 2008. A Systematic Approach for Evaluation of Capture Zones at Pump and Treat Systems. Ada, OK: U.S. Environmental Protection Agency, National Risk Management Research Laboratory. https://cfpub.epa.gov/si/si_public_record_report.cfm?Lab=NRMRL&dirEntryId=187788. ). This guidance promotes developing “converging lines of evidence,” by applying multiple techniques to evaluate capture and increase confidence in the conclusions of capture analysis ( USEPA 2008[7ILB4T4V] USEPA. 2008. A Systematic Approach for Evaluation of Capture Zones at Pump and Treat Systems. Ada, OK: U.S. Environmental Protection Agency, National Risk Management Research Laboratory. https://cfpub.epa.gov/si/si_public_record_report.cfm?Lab=NRMRL&dirEntryId=187788. ). The multiple lines approach is also recommended for evaluating other performance evaluating criteria.
When evaluating plume containment by extraction, impacts from nearby water supply or irrigation wells and other water-processing facilities (e.g., quarries) and impacts from groundwater mounding by stormwater management also need to be considered as possible temporal stressors both on site and off site. Water supply wells including private and irrigation wells and public water supply wells are not part of the extraction system, but operation of these wells may have a negative impact on groundwater extraction efficiency or even cause failure of the remedy. For example, excessive pumping from a water well may change groundwater flow direction locally and alter both contaminant migration and the capture zone, potentially resulting in a lack of capture. Private wells are sometimes drilled using methods that do not account for the need to provide a seal between water-bearing zones and may serve as conduits between different aquifers. This may promote contaminant migration between aquifers and can cause failure of the remedy. Therefore, evaluation of the P&T system should also include monitoring of nearby water supply and irrigation wells. If operating conditions of these wells change, an updated plume capture analysis may be warranted to understand the effects of these changes on the P&T system and to avoid failure of plume containment.
If inadequate plume capture is identified, the P&T extraction system may need to be augmented by increasing pumping rates from wells and/or adding more strategically located extraction wells. Quantitative analysis of plume capture can be conducted with properly calibrated models, although it is important to understand that models have limitations and a certain level of uncertainty relevant to efforts to produce a unique solution. (See Appendix A. Common Concepts, Section 2.1 Modeling Limitations) Particle tracking in conjunction with a calibrated groundwater flow model is widely used at P&T sites to evaluate hydraulic capture. A general discussion of modeling is provided below (Section 3.3.3) and in Appendix A. Details for P&T system optimization are discussed in Section 4.3.1.3.
3.3.1.1.3 Evaluating NAPL Removal
Although P&T alone is not very effective at NAPL removal (including dense nonaqueous-phase liquid (DNAPL) and light nonaqueous-phase liquid (LNAPL)), it could be a component of NAPL removal technologies like product skimming and MPEs. NAPL removal from the source area by other technologies is normally needed before implementation or full effectiveness of P&T. ITRC has guidance documents for NAPL removal or NAPL remedial technologies (e.g., ( ITRC 2009[CI993T5P] ITRC. 2009. “Evaluating LNAPL Remedial for Achieving Project Goals.” Washington D.C.: Interstate Technology & Regulatory Council, LNAPL Team. https://www.itrcweb.org/GuidanceDocuments/LNAPL-2.pdf.ITRC 2020[JWKDF22N] ITRC. 2020. “Integrated DNAPL Site Strategy.” Washington D.C.: Interstate Technology & Regulatory Council, IDSS Team. https://idss-2.itrcweb.org/. )), and this section focuses only on NAPL removal related to P&T technology. Evaluation of extraction effectiveness should address NAPL removal efficiency from extraction wells if NAPL removal is one of the remedy goals for the P&T system. If a significant drop in NAPL removal efficiency is observed, well locations, pump positions, and pump types need to be evaluated and adjusted as necessary to improve NAPL removal efficiency. The feasibility of continuous NAPL removal with P&T should also be evaluated and termination of the operation is warranted if continuous NAPL removal with P&T becomes impractical. If NAPL is identified after the extraction system was installed and NAPL removal is determined to be necessary and feasible by P&T, an NAPL data gap evaluation will need to be completed. The CSM would need to be updated accordingly, and the P&T system may need to be updated. The goal of the Performance Based Optimization of Pump & Treat Systems Guidance is not to focus on NAPL removal but simply state that some P&T systems may have to deal with this situation. For additional information on LNAPL, go to the ITRC website at https://lnapl-3.itrcweb.org/.
3.3.1.1.4 Evaluating Groundwater Quality Restoration
If groundwater cleanup and restoration to a specific RAO is the goal of the P&T remedy, a system performance evaluation should be completed to determine whether this remedial objective is still achievable in a reasonable time frame. Most often, P&T systems alone are not effective in fully restoring the groundwater quality, especially when the source of the COCs in groundwater has not been removed. Other active treatment technologies like bioremediation, stabilization, chemical oxidation, chemical reduction, and thermal remediation are often implemented in combination with P&T to treat or remove contaminant mass from these source zones and other high concentration areas.
If the P&T remedy achieves the groundwater quality RAO under pumping conditions, the RAO may still be exceeded after the termination of pumping as a result of the concentration rebound caused by the matrix back-diffusion effects. A rebound test, during which the concentrations are monitored both prior to and after the cessation of pumping, can help project the time needed to meet the RAOs. Rebound testing is explained in detail in Section 3.7 of Appendix A.
If the evaluation determines that achievement of this remedial goal is technically impractical (TI) with available remedy technologies (e.g., due to the anticipated unreasonably long restoration time frame, presence of NAPL, low-permeable layer, or fractured rocks), the RAOs need to be updated, and a TI waiver for achieving the ARARs may be applied. Details on how to evaluate TI for groundwater restoration can be found in USEPA 1993 guidance ( USEPA 1993[K447EQCV] USEPA. 1993. “Guidance for Evaluating the Technical Impracticability of Ground-Water Restoration.” U.S. Environmental Protection Agency, Office of Solid Waste and Emergency Response. https://nepis.epa.gov/Exe/ZyPDF.cgi/100021PJ.PDF?Dockey=100021PJ.PDF. ) or the state’s applicable TI guidance; the topic is discussed in Section 7.4.1. Hydraulic containment may still need to be maintained by continuous or intermittent pumping at certain extraction wells to ensure that the plume migration is controlled outside of the TI zone. If the evaluation determines that groundwater restoration will not be practically achieved by P&T but can be achieved through other remedies, transition out of P&T will be considered. More details about transitioning will be discussed in Section 5.
3.3.1.2 Evaluation of Performance of Monitoring Wells
Monitoring wells provide crucial data to evaluate and potentially improve the performance of a P&T system. Contaminant concentrations at sentinel and compliance monitoring wells indicate efficiency of plume containment and provide early warning of potential exposure to downgradient receptors. Therefore, monitoring wells need to be designed to intercept the plume, and the number and locations of the wells need to be suitable to provide adequate information to determine plume migration trends and plume containment efficiency. Subsequently, the wells need to be assessed and maintained to ensure that they can provide representative data for plume evaluation.
The evaluation of monitoring wells should include assessment of their construction details (e.g., well depth, screened interval as related to the stratigraphy), current physical condition (e.g., casing damage, debris, grout intrusion) and the history of being dry. It should also include evaluations of sampling frequency and adequacy of the monitoring parameters. An efficient monitoring-well network should have optimized well locations and well numbers in key plume areas (e.g., background; source area; in the center of the plume, in a lateral part of the plume, or near the plume boundary; and in sentinel and compliance positions). The number of performance monitoring wells should be sufficient to fully delineate and monitor the plume. The United States Department of the Navy has guidance for planning and optimizing monitoring strategies, including designing and optimizing the monitoring-well network and locations and determining and optimizing monitoring duration and frequency ( NAVFAC 2010[SWFQZYK4] NAVFAC. 2010. “Department of the Navy Guidance for Planning and Optimizing Monitoring Strategies.” U.S. Department of Defense, Department of the Navy. https://frtr.gov/matrix/documents/Monitored-Natural-Attenuation/2010-Guidance-for-Planning-and-Optimization-of-Remedial-Strategies.pdf. ). Additional discussion is presented in Section 3.3.4.
3.3.1.3 Statistical Tools
Analytical and statistical tools for both extraction effectiveness and evaluation of trends are important for P&T system development and performance evaluation. These tools assist in evaluating the contaminant concentration trends, evaluating contaminant mass discharge, and predicting future plume behavior to demonstrate the effectiveness of the P&T system. ( Truex et al. 2017[3HUEBEH4] Truex, Michael, Chris Johnson, Tamzen Macbeth, Dave Becker, Kira Lynch, Dominic Giaudrone, Aaron Frantz, and Hope Lee. 2017. “Performance Assessment of Pump-and-Treat Systems.” Groundwater Monitoring & Remediation 37 (3): 28–44. https://doi.org/https://doi.org/10.1111/gwmr.12218.Truex et al. 2015[VKN225ST] Truex, M.J., C.D. Johnson, D.J. Becker, M.H. Lee, and M.J. Nimmons. 2015. “Performance Assessment for Pump and Treat Closure or Transition.” Pacific Northwest National Laboratory. https://www.pnnl.gov/main/publications/external/technical_reports/PNNL-24696.pdf. ).
Contaminant concentration trends are used to evaluate whether the concentrations within the plume have remained stable, increased, or declined during the P&T operations. These trends are evaluated using concentration versus time graphs at monitoring and extraction wells. A comprehensive review of the types of trend analysis methods is provided in Appendix A (Common Concepts). The applications and limitations of temporal trend analysis are discussed in the ITRC’s 2013 document Groundwater Statistics and Monitoring Compliance and ITRC’s 2019 webinar Groundwater Statistics for Environmental Project Managers ( ITRC 2014[23XY7CSP] ITRC. 2014. “Groundwater Statistics for Environmental Project Managers.” Groundwater Statistics for Environmental Project Managers Sponsored by: Interstate Technology & Regulatory Council. 2014. https://clu-in.org/conf/itrc/gsmc/.ITRC 2013[4DY4TN8V] ITRC. 2013. “Groundwater Statistics and Monitoring Compliance.” Washington, D.C.: Interstate Technology & Regulatory Council, Groundwater Statistics and Monitoring Compliance Team. https://projects.itrcweb.org/gsmc-1/. , Truex et al. 2017[3HUEBEH4] Truex, Michael, Chris Johnson, Tamzen Macbeth, Dave Becker, Kira Lynch, Dominic Giaudrone, Aaron Frantz, and Hope Lee. 2017. “Performance Assessment of Pump-and-Treat Systems.” Groundwater Monitoring & Remediation 37 (3): 28–44. https://doi.org/https://doi.org/10.1111/gwmr.12218. ). Readily available software applications can perform temporal trend analysis and are useful tools for evaluating P&T effectiveness. A summary of the most commonly used software is presented in Appendix A (Common Concepts).
Spatial moments analysis is a Lagrangian approach for evaluating and monitoring the contaminant mass through both space and time. The first three spatial moments (zeroth, first, and second) assess the dissolved phase mass, solute velocity, and dispersion of the contaminant plume ( Govindaraju and Das 2007[J6NE9T9W] Govindaraju, Rao S., and Bhabani S. Das. 2007. “Moment Analysis for Volatile Compounds.” In Moment Analysis For Subsurface Hydrologic Applications, 155–82. Dordrecht: Springer Netherlands. https://doi.org/10.1007/978-1-4020-5752-6_7. ). The zeroth moment is an integrated measure of the mass within a spatial extent at a specific snapshot in time. The first moment provides an estimate of the mean solute velocity and can be used to evaluate the rate of movement of the center of mass of the plume. The second moment provides an estimate of the dispersion, or spread, of the plume. A review of spatial moments methodologies and readily available software for calculating spatial moments is presented in Appendix A (Common Concepts).
Several case studies (Appendix B) highlight concerns related to the evaluation of extraction system and monitoring wells that impact assessment of performance. Use of existing operational and monitoring data and collection of supplemental data led to modifications of the extraction and/or monitoring approach for the following cases:
- Michigan P&T Case Study—Review of potentiometric maps and chemical trends did not support the notion that the P&T remedy was achieving hydraulic and chemical capture. This included an evaluation of existing data, trend analyses of the downgradient monitoring-well network, an assessment of aquifer-plume intercept effectiveness, and an assessment of well hydraulics (pumping rates) with possible additional wells and groundwater model.
- McGaffey and Main, New Mexico—An optimization review was implemented in 2015 that included P&T as a method for hydraulic containment. This optimization effort focused on in situ methodologies, but P&T was used for hydraulic containment. Alternatively, enhanced reductive dechlorination (ERD) would have been implemented if this hydraulic control system was not practical due to concerns regarding water rights constraints. As part of this optimization review, which included several in situ alternatives (i.e., ISCO, in situ thermal treatment (ISTT), ERD), assessment of sources within the soils was conducted to facilitate plume core mass reduction. Operation of the extraction system was paused to collect this additional information. After the source is reduced to acceptable levels, groundwater P&T containment (part of the overall remedy) may be refined to serve as a component of a preferred alternative, assuming water rights are not an ongoing constraint. This case study shows how integration of monitoring and an existing hydraulic control system can be a useful component of an implemented remedy.
3.3.2 Injection and Recirculation of the Treated Water
P&T systems may include either injecting the treated water into the aquifer as a means of discharging or creating a recirculation zone for flushing of the COCs in the source zone. When effluent will be discharged, whether for injection or recirculation, it should be analyzed prior to discharge, and the results should be used to assess P&T system effectiveness and for compliance with any relevant aquifer-intercept zone compatibility concerns, applicable regulatory requirements, and associated permits. The evaluation of the performance of the injection/recirculation system is discussed in this section.
3.3.2.1 Evaluation of Treated Water Injection
At many P&T sites the treated water is discharged back into the subsurface through injection wells or infiltration galleries. This may be done as the most expedient way of discharging the treated water, to flush the source area in the saturated or vadose zone and thus accelerate the COC mass removal, or to flatten the hydraulic gradient in the vicinity of extraction wells and increase the capture zone radius of influence near the extraction wells, potentially reducing the overall extraction rate ( USEPA 2009[8F6DQD9W] USEPA. 2009. “Green Remediation Best Management Practices: Pump and Treat Technologies.” U.S. Environmental Protection Agency, Office of Solid Waste and Emergency Response. https://semspub.epa.gov/work/HQ/147210.pdf. ).
Like the extraction wells, the injection wells and infiltration galleries should undergo routine maintenance to manage screen/sand pack clogging and ensure adequate capacity. Typically, injection wells are more susceptible to fouling than extraction wells for multiple reasons such as increased oxygen levels in the injected water (due to pressure and treatment processes) and other geochemical/microbial incompatibility characteristics. If well rehabilitation (See Section 4.3.1.6) does not restore the capacity of the injection wells to the target values or the capacity of the existing injection well system will not take all the treated water, new injection wells may need to be installed. In addition to routine maintenance of the injection wells, the impact of the injected water, subsurface hydrology, and aquifer geochemistry should be evaluated during the design phase. Such evaluations should generally occur before the installation of the P&T system. If potential adverse effects are detected, other discharge options (e.g., to surface water, to POTW, etc.) should be considered.
3.3.2.2 Evaluation of Recirculation
Recirculation in a P&T system involves injecting or infiltrating treated effluent from the treatment system into the subsurface so the introduced water is recaptured by the P&T system. Recirculation can be intentional (explicitly designed as part of the remediation system) or unintentional. Intentional recirculation is generally done to enhance the performance of a groundwater remediation system, as follows:
- increasing flushing of the plume area with treated water
- providing groundwater for introducing amendments or treatment chemicals to the dissolved groundwater plume
- reducing the volume of groundwater discharged by the P&T system to other discharge options, such as POTW or surface water
- recirculating for water conservation objectives or to comply with water rights and potentially avoid costs associated with acquiring water rights and/or associated replenishment fees
Recirculation can be implemented to enhance remediation using either horizontal or vertical groundwater flow. With a horizontal configuration, treated or amended water is introduced upgradient of the pumping wells to circulate water horizontally through the treatment zone of the aquifer in the path of groundwater flow into the extraction wells. The treated water can be injected via wells, infiltrated via discharge across the land surface, or discharged into an engineered basin or pond or to an infiltration gallery. With a vertical configuration, treated or amended water is introduced in a position vertically above and upgradient of the position of the well screen of the recovery well; the water moves vertically downward in the aquifer to create a zone of vertical circulation in the immediate vicinity of the recovery well.
3.3.2.2.1 Intentional vs. Unintentional Recirculation
As described above, intentional recirculation can be implemented with the objective of improving the P&T system’s performance. Unintentional circulation is the discharge to a point believed to be downgradient of the P&T system’s capture zone, but which actually is within the capture zone. The unintentional recirculation may compromise the system’s performance by introducing unintended flow into the capture zone and thus decreasing its size.
A detailed CSM is vital when evaluating recirculation used as a component of a P&T system. Advanced Site Characterization Tools (See ITRC fact sheet on Implementing the Use of Advanced Site Characterization Tools ( ITRC 2019[ZMM54HEL] ITRC. 2019. “Implementing Advanced Site Characterization Tools.” Washington, D.C.: Interstate Technology & Regulatory Council, Implementing Advanced Site Characterization Tools Team. https://asct-1.itrcweb.org/. )) are valuable for collecting detailed data to assess the effectiveness of recirculation. Evaluation of the performance of a P&T system explicitly designed to include recirculation involves reviewing multiple types of data, including the following:
- water levels, hydraulic gradients, and resulting groundwater flow directions to determine whether groundwater movement is in the desired directions
- geochemical compatibility characteristics of injectate/infiltrate (treater water) within the aquifer system (i.e., each hydrostratigraphic unit) and aquifer-intercept zone (that may also include/impact microbiological)
- COC concentrations within the groundwater plume to determine the effectiveness of the remediation system
- concentrations of amendments or chemical reagents used for in situ groundwater plume/source treatment
- volumes of water injected, pumped, treated, and discharged (if not all is being recirculated) to allow calculation of a water balance for the system and determine what percentage of the injected water is being recovered
Collectively, these data are used to evaluate the performance of the recirculation system by assessing whether the following factors are true:
- capture zones for the P&T system are sufficient to recover the injected/infiltrated water
- concentrations of COCs within the plume area exhibit decreasing trends that are generally similar to those forecast by groundwater and/or geochemical modeling
- amendments added to the injected/infiltrated water are at sufficient concentrations to treat the COCs to the desired levels
- mixing of the injected water and the impacted groundwater in the treatment area creates undesired geochemical changes in the subsurface
Evaluation of unintentional recirculation relies on much of the same data as evaluation of intentional recirculation. However, monitoring data where unintentional recirculation is occurring may not be as comprehensive as that available with intentional recirculation since the unintentional recirculation was not anticipated during planning of the monitoring program.
The evaluation of both intentional and unintentional recirculation is more effective when data interpretations are combined with the use of groundwater models (See Section 3.3.3). Groundwater models can be used to simulate capture zones for the pumping wells and calculate groundwater flow paths between the injection/infiltration areas and the pumping wells. Geochemical models can be used to evaluate changes in geochemistry along the groundwater flow paths to improve the understanding of potential interactions between the injected/infiltrated water and the impacted groundwater.
3.3.3 Groundwater Flow and Transport Modeling
Models enable complex hydrogeologic systems to be represented for purposes of evaluating physical and chemical behaviors during performance evaluation (e.g., extraction, injection/recirculation, plume containment, plume migration). Modeling can significantly improve the understanding of a site’s CSM and can be used to predict future performance while incorporating uncertainty analysis ( Anderson, Woessner, and Hunt 2015[SSSNUX95] Anderson, M., W. Woessner, and R. Hunt. 2015. Applied Groundwater Modeling, Simulation of Flow and Advective Transport. 2nd ed. Elsevier. ). Groundwater flow predictions (e.g., water level drawdown, capture zone, etc.), which rely on groundwater flow models, have a narrower band of uncertainty when compared to contaminant transport forecasts, which rely on both groundwater flow and transport models, effectively compounding uncertainty associated with both models. Estimating groundwater cleanup times is even more complicated since, in many cases, issues such as back diffusion of contaminants from low permeability zones can dominate the time to restore aquifers ( Borden and Cha 2021[ZZ99ATMC] Borden, R.C., and K.Y. Cha. 2021. “Evaluating the Impact of Back Diffusion on Groundwater Cleanup Time.” Journal of Contaminant Hydrology, no. 243: 103889. ).
Groundwater models can be used to more effectively close data gaps through uncertainty analysis, evaluate cost benefits associated with revisions to P&T systems, and help quantify performance uncertainties that can influence future costs. Groundwater models can also be used for other risk-management evaluations such as the potential for differential subsidence on existing structures/infrastructure due to dewatering or lowering of hydraulic heads. For example, modeling was used to evaluate the existing P&T system at the Saco Defense site (see Appendix B, Case Studies), as well as to compare it to possible enhancements. These evaluation studies showed that enhancing the existing system would not significantly reduce the remediation time frame but would increase costs.
Modeling is an important tool for P&T system development and evaluation, but as with any tool its use must be tempered with need, data availability, and budget. There are several different levels of modeling complexity and cost, and the cost of performing modeling increases with model complexity. The tenets of model parsimony are described by many authors, and publications such as A Decision Framework for Minimum Levels of Model Complexity (National Ground Water Association ( NGWA 2017[46PV3JH8] NGWA. 2017. “White Paper on Groundwater Modeling.” National Groundwater Association. https://my.ngwa.org/NC__Product?id=a183800000uwvpaAAA. )) may be consulted to assist in development of an optimized modeling program. Within these limitations, P&T models should be updated as needed to incorporate new data and improved understanding of the CSM. Appendix A (Common Concepts) contains additional details on modeling P&T systems. Other resources include guidance on capture analysis such as the USEPA’s A Systematic Approach for Evaluation of Capture Zones at Pump and Treat Systems ( USEPA 2008[7ILB4T4V] USEPA. 2008. A Systematic Approach for Evaluation of Capture Zones at Pump and Treat Systems. Ada, OK: U.S. Environmental Protection Agency, National Risk Management Research Laboratory. https://cfpub.epa.gov/si/si_public_record_report.cfm?Lab=NRMRL&dirEntryId=187788. ). Modeling is further discussed in Section 4.3.1.3 for use in P&T system optimization.
Appendix B includes the case study for Saco Defense, Inc., in Maine and Bob’s Industrial Recycling facility in Michigan; both case studies highlight the use of modeling during evaluation efforts.
3.3.4 Extraction-Well and Monitoring-Well Network Considerations
Extraction and monitoring wells are integral parts of every P&T system. The ability of the extraction wells to withdraw groundwater is essential to achieving typical objectives of P&T, such as obtaining plume containment and providing recovery of the COC mass. The system’s achievement of these objectives is evaluated using information provided by the monitoring-well network and the extraction-well data. The focus of the Performance Based Optimization of Pump & Treat Systems guidance is on extraction, monitoring, and plume control using the well network. Although injection wells and the injection gallery are not a major focus of this guidance, Section 4 and other sections address key aspects of injection well use and optimization. For a more extensive explanation of injection systems refer to ITRC’s Optimizing Injection Strategies and In situ Remediation Performance guidance document ( ITRC 2020[SKNUVGR8] ITRC. 2020. “Optimizing Injection Strategies and In Situ Remediation Performance.” Washington, D.C.: Interstate Technology & Regulatory Council, OIS-ISRP Team. https://ois-isrp-1.itrcweb.org/. ), which extensively covers amendment selection considerations, amendment delivery optimization, and the monitoring-well network; the document includes Appendix D. Injection Fact Sheets.
This section presents an overview of factors to consider when evaluating the performance of the monitoring and extraction wells. It includes a brief discussion of the testing of extraction-well efficiency as part of the P&T system performance evaluation. It also provides a discussion of performance assessment under complex subsurface conditions.
Gaining an appropriate level of knowledge about the extraction system and its extent of influence horizontally and vertically supports evaluation of its effectiveness in controlling gradients and capturing groundwater impacted by COCs. One best approach to this is having monitoring wells (and/or piezometers) installed at various depths corresponding to or addressing known heterogeneities and boundaries within the aquifer. Having a monitoring network (of wells and piezometers) that effectively represents heterogeneities, boundary conditions, and gradients (hydraulic and vertical potential) that can be influenced during pumping—to a relevant and appropriate level of understanding—should support assessment of remedial objectives. Knowing that the existing monitoring network of wells and piezometers represents reasonable locations and depths and that these represent heterogeneities or boundaries that can affect performance facilitates collection of sufficient data to address performance data gaps.
The evaluation of the performance of the extraction and monitoring wells is a key step in the optimization of the P&T system. The subsequent steps include further actions related to the extraction-well and monitoring-well networks. Section 4 presents a discussion related to optimization, including the implementation strategies (See Sections 4.3 and 4.4). Section 5 considers transition strategies, and Section 6 discusses the issues related to the sustainability and resiliency of the system.
3.3.4.1 Monitoring Wells
Performance assessment of P&T remedies requires monitoring of the system performance against remedial objectives. As discussed in Section 2.1.1, P&T remedies are selected for a combination of three objectives: source containment/management, plume hydraulic containment, and aquifer restoration. If source containment is one of the objectives of the remedy, monitoring should be designed to assess effective containment of releases from source area(s) focusing on the hydraulic gradients and contaminant concentrations. If P&T is selected to provide hydraulic containment of the plume within a boundary, the monitoring network should focus on evaluating hydraulic conditions around the boundary. These two properties are used to demonstrate that plume capture is effective as designed and protective of human health and the environment. To assess aquifer restoration, the monitoring should be distributed over the entire area targeted for restoration.
A performance monitoring program at a P&T site should include the hydraulic and contaminant monitoring necessary to evaluate the success of the remedial action.
Hydraulic monitoring of a P&T remedy is required to evaluate capture effectiveness and supports the evaluation of subsurface contaminant conditions. Since hydraulic stresses typically are more homogeneous than contaminant concentrations in an aquifer, the typical well network needed to collect hydraulic data is less dense and more regularly spaced than a contaminant monitoring-well network. In cases where heterogeneity can more directly influence the distribution of hydraulic stresses within an aquifer, such as fracture-conduit flow in karst or typically heterogeneous and anisotropic conditions associated with fluvial depositional systems, collection of hydraulic head data focused on transmissive zones or localized areas of greater specific storage may provide better representative data to support evaluation. Remote sensing techniques coupled with intrusive field screening / exploratory methods can facilitate targeting these subsurface features prior to installation of additional monitoring points, piezometers, or additional monitoring wells. The network should extend beyond plume boundaries. The rationale for determining the locations for these wells includes focusing around the margins of the plumes (confirming/evaluating inward or reduced gradients), in the core of the plumes near the extraction wells (to collectively identify the magnitude and shape of the groundwater depression caused by pumping), and in the areas where general flow directions in the aquifer can be identified and monitored over time. In addition, installation of piezometers paired with extraction wells allows a better representation of aquifer drawdown to be obtained than measuring water level directly in an extraction well. This is because measuring directly in the extraction wells cannot distinguish between the aquifer drawdown, which is the target of the hydraulic monitoring, and the well losses.
Hydraulic monitoring networks sometimes use transducers and data loggers to measure groundwater elevations for specific needs, but most of these data are collected during routine sampling events. Collecting data more frequently in areas or zones where moderate to large changes in head caused by pumping or regional stresses can be critical in understanding inconsistencies in operational and monitoring trends. These data collection needs are best served by the installation of pressure transducers / data loggers. Short-term or long-term installation of these instruments, periodically downloaded for analysis of trends, can be a cost-effective way to collect these sequential and frequent data points that will support assessment of more dynamic performance trends. Having these as baseline data will support refinement of the CSM and will justify optimization considerations. Data collected during routine sampling events is sufficient for areas less affected by these dynamic conditions. Data collected provide useful model calibration data for the remedy and allow for the development of capture frequency maps for the approximate extent of hydraulic containment. Furthermore, for the areas where aggressive pumping is needed (e.g., areas with higher contaminant concentrations), this monitoring would support evaluating the sustainability of the extraction field by optimizing pumping and upgradient and downgradient injection.
The hydraulic monitoring network is expected to evolve as the subsurface and plume conditions change during the lifetime of the remedy. Some level of hydraulic monitoring will still be needed following the cessation of the active treatment to evaluate the return of hydraulic gradients to a more regional groundwater flow pattern.
Contaminant monitoring data are collected over the lifetime of the P&T remedial action. Data collection primarily relies on a monitoring-well network as well as data collected at extraction wells to evaluate the subsurface conditions. Collectively, data are used to evaluate system performance against the functional objectives of the P&T system, optimize effectiveness, and determine whether the remedial action is complete. Following the initial design, the monitoring-well network is expected to evolve as the subsurface conditions change during the lifetime of the remedy (e.g., groundwater levels, plume distribution, etc.). Some plume areas may reach cleanup standards more quickly than others. In these areas, extraction wells will likely be shut down in stages as contaminant concentrations are reduced and their contributions to hydraulic containment become less important. Rebound studies are typically conducted at these stages. Similarly, the monitoring-well network may also evolve due to transient hydraulic conditions in the subsurface (e.g., wells may go dry).
It is important to ensure that spatial coverage of the monitoring-well network, both laterally and vertically, is consistent with the current understanding of the plume distribution within the boundaries of the targeted hydraulic containment zone. A denser horizontal and vertical coverage is typically needed around the higher concentration portions (typically along plume axes) and the leading edge of the contaminant plumes as well as in the vicinity of potential continuing sources and receptors or when interbedded geology is identified. As site conditions change and the CSM is updated, a systematic process is needed to evaluate and identify data gaps in monitoring well coverage. This process may combine spatial statistics with an evaluation of hydraulics and plume distribution using a fate and transport modeling approach. Some factors that would need to be considered for a data gap analysis include proximity to active extraction wells, kriging error variances for plumes, capture frequency predictions, and predicted concentrations over time. The process of data gap analysis overlapped with the uncertainties associated with the CSM yields a prioritized list of potential monitoring well locations and identifies where additional coverage is needed or where redundant coverage exists. In addition to the locations of the monitoring wells, monitoring frequency at each well should be determined. Determining the data collection frequency depends on the monitoring objectives. For example, where highly transient locations or zones may require more frequent monitoring approaches, monitoring frequency can be reduced for the areas that have achieved steady-state conditions. For more information on monitoring optimization, see Section 4.3.2.
3.3.4.2 Extraction Wells
During system design, the extraction rate of a well is typically calculated using the properties of the aquifer in which the well will be screened (e.g., hydraulic conductivity, thickness of the saturated zone, recharge, presence of hydrogeologic boundaries). In many cases, however, the actual extraction rates are lower than the rates thus estimated, even though a factor of safety is typically used during the design, and do not meet the design objectives. This is a common problem in P&T systems. It is caused by the high energy loss occurring in the extraction well’s screen and filter pack. This may be the result of design and installation issues, such as damage and plugging of the aquifer during drilling, inappropriate filter pack design, inadequate well diameter, or insufficient open area of the screen. It can also be caused by plugging of the filter pack and screen, or surrounding formation, during the operation due to biochemical processes (e.g., corrosion, mineral deposition, or biomass biofouling associated with microbial activity).
To achieve the project objectives, such as hydraulic containment of the dissolved plume or mass removal, the extraction well(s) must be maintained in an acceptable flow range. It is important to evaluate the flow at startup to obtain the baseline by which the well performance is assessed during the operation of the system because the performance is typically best following the well installation and development and declines with time as the well experiences fouling. Routine well efficiency evaluation can inform the maintenance schedule (e.g., well redevelopment, well cleaning) and help maintain the optimum well performance. Measures to rehabilitate extraction wells are further discussed in Section 4.3.1.6.
Performance of an extraction well is typically assessed using the concepts of well efficiency or well specific capacity (illustrated on Figure 3‑1) or by indirect methods. The assessment methods are discussed below.
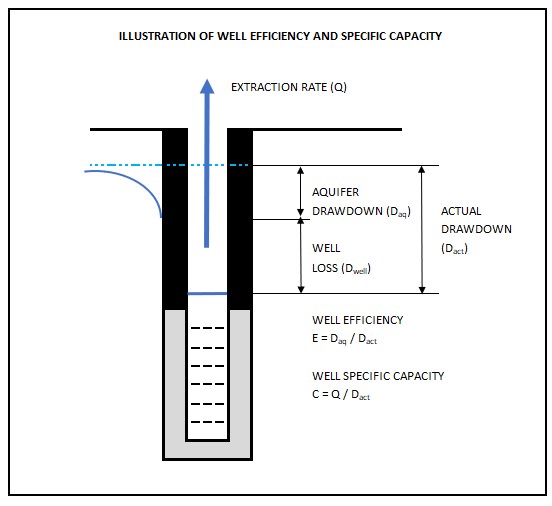
Source: M. Ostrowski. Used with permission.
Note that, in addition to the loss of well efficiency, flow reduction can also be a result of depletion of aquifer storage as evidenced by the decrease of the ambient potentiometric levels. This can be recognized by examining the results of the hydraulic monitoring (Section 3.3.4.1.)
3.3.4.2.1 Well Efficiency
Total drawdown observed in the pumping well during operation consists of two components: aquifer loss and well loss. Aquifer loss is proportional to the flow rate from the aquifer to the outer face of the well, and well loss is the loss of energy as the groundwater flows through the sand pack and screen. Well loss is not linear and increases rapidly as the flow rate increases. The efficiency of a pumping well expresses the ratio of the aquifer drawdown (theoretical drawdown) to the actual (measured) drawdown in the well, at a specified flow rate ( Kruseman and de Ridder 1990[X7XM6LWU] Kruseman, G.P., and N.A. de Ridder. 1990. Analysis and Evaluation of Pumping Test Data Second Edition (Completely Revised). Vol. ILRI Publication 47. Wageningen, Netherlands: International Institution for Land Reclamation and Improvements. ). This is illustrated in Figure 3‑1.
As an example, if the well efficiency is determined to be 0.3, the extraction rate is driven by 30% of the observed well drawdown, and the remainder of the observed well drawdown (70%) is due to the energy losses across the well screen and sand pack.
Well efficiency can be determined by performing a step drawdown test in which the well is pumped at several rates (at least three steps) while measuring total drawdown in the well. At each step, the pumping continues until a stable drawdown is achieved or sufficient data are available to extrapolate the time-drawdown curve to estimate the stable drawdown. Drawdown and extraction rate data pairs are analyzed by means of regression or trial-and-error to determine the aquifer drawdown and well losses.
Another method of determining the well efficiency is to install a piezometer immediately outside of the extraction wells and monitor the hydraulic heads in the extraction well and the piezometer. Readings collected in the piezometer provide the hydraulic head at the outer face of the extraction well, which allows the aquifer drawdown to be estimated, while the readings inside the well provide the actual drawdown. The well efficiency can be calculated directly from these readings.
3.3.4.2.2 Well Specific Capacity
The specific capacity is the ratio of the extraction well’s steady-state pumping rate and the corresponding steady-state drawdown in the well. It is determined by extracting groundwater from the well at a constant rate until a stable drawdown and flow can be achieved or sufficient data are available to extrapolate the data to obtain these parameters. The extraction rate is then divided by the drawdown to obtain the specific capacity. This is illustrated on Figure 3‑1.
3.3.4.2.3 Indirect Methods
Indirect methods of assessing well efficiency include analyzing the O&M records to identify declining extraction rates (e.g., flow records, pumping rates through time, pump down time, and pump cavitation). When the well performance, assessed using any of the abovementioned methods, declines below the criteria established for the project, maintenance activities must be performed (see Section 4.3.1.6). Geochemical and microbial data analysis, removal and evaluation of in-well equipment (e.g., submersible pumps and piping), or downhole videos of extraction wells are beneficial for conducting root cause analysis. It is important to note that conditions related to the degradation of well performance within the well and surrounding aquifer can, and commonly do, extend up into the conveyance system, and possibly further into the equalization and treatment processes. Changes in conveyance hydraulics due to accumulations of biomass, mineral incrustation, and sediment deposition alter pipe flow, velocities, and head losses, which can lead to restrictive flow conditions and eventually air lock conditions if no corrective actions are taken (see Section 4.3.3.5 for a more detailed discussion on enhancements to the existing P&T system).
3.3.4.3 Complex Hydrostratigraphic Conditions
The spatial distribution (both vertical and horizontal), density, and construction of the extraction-well and monitoring-well network are critical components of a successful P&T system and must be considered when complex subsurface conditions exist at a site. Numerous monitoring wells are likely necessary to identify and address aquifer characteristics, such as the following:
- site heterogeneities in aquifer matrix characteristics (e.g., grain size, transmissivity, and storativity values)
- presence of fractured bedrock
- local and regional hydrostratigraphy including aquitards and aquicludes
- boundary conditions (natural, anthropogenic/infrastructure, engineered, and previous remedies)
- groundwater chemistry, concentrations, and plume/source morphology (including commingled plumes of differing chemicals)
- presence of NAPLs
Multiple subsurface conditions may also trigger additional regulatory requirements. For example, some states regulate or restrict the use of well screens across multiple water-bearing zones, and some states dictate well construction (e.g., double casings) for wells installed in or through contaminated aquifers.
In the process of evaluating the performance of a P&T system, the construction of the extraction-well and monitoring-well network must be considered when multiple subsurface conditions exist at a site. Example considerations include the use of head data from wells that are screened in a different stratigraphic zone than an extraction well being evaluated or evaluating recovery from long-screen extraction wells when the CSM indicates discrete contaminant interval(s) or multiple stratigraphic zones.
3.3.5 Evaluation of the Treatment System
Detailed discussion of the evaluation of the treatment system is beyond the scope of this report, and only a brief outline is provided here. A good description of evaluating a treatment system can be found in Above Ground Treatment Technologies ( ITRC 2006[7FD9Z567] ITRC. 2006. “Above Ground Treatment Technologies.” Washington, D.C.: Interstate Technology & Regulatory Council, Remediation Process Optimization Team. http://itrcweb.org/Guidance/GetDocument?documentID=82. ).
Whether discharging to a municipal sewer system, surface water body, or to the ground or infiltration gallery, local or state regulations usually have well-defined discharge limits. These limits may be imposed on the concentrations of the individual COCs, the volume discharged, or the bulk contaminant loads.
Performance assessments are crucial to determining the effectiveness of a treatment system and assessing its ability to meet the project discharge limits (see Section 5.3.1) Performance is evaluated based on monitoring the flow rate entering the treatment system and on the influent and effluent samples collected for analysis for the regulated COCs. The sampling and monitoring are performed on a predetermined schedule. The data are then compared to the project discharge limits.
If the discharge limits are not being met, the functioning of the treatment system should be evaluated to identify the corrective measures needed. A wide variety of treatment options are available for the extracted groundwater; the best option(s) will depend on the groundwater chemistry, COCs, discharge requirements, etc., resulting in multiple performance evaluation methodologies. The description of this type of analysis is not in the scope of this document (see Above Ground Treatment Technologies ( ITRC 2006[7FD9Z567] ITRC. 2006. “Above Ground Treatment Technologies.” Washington, D.C.: Interstate Technology & Regulatory Council, Remediation Process Optimization Team. http://itrcweb.org/Guidance/GetDocument?documentID=82. )).
Even if discharge limits are being met, the system should be carefully monitored for signs of decreasing performance that may lead to exceedances in the future (e.g., back pressure increases). Periodic evaluations should also include a review of the costs associated with the P&T system. A cost/benefit analysis should look at where money is being allocated ineffectively (e.g., operations, labor, utilities, consumables). Figure 3‑2 shows one example of a flow diagram that can be used to evaluate your treatment system.
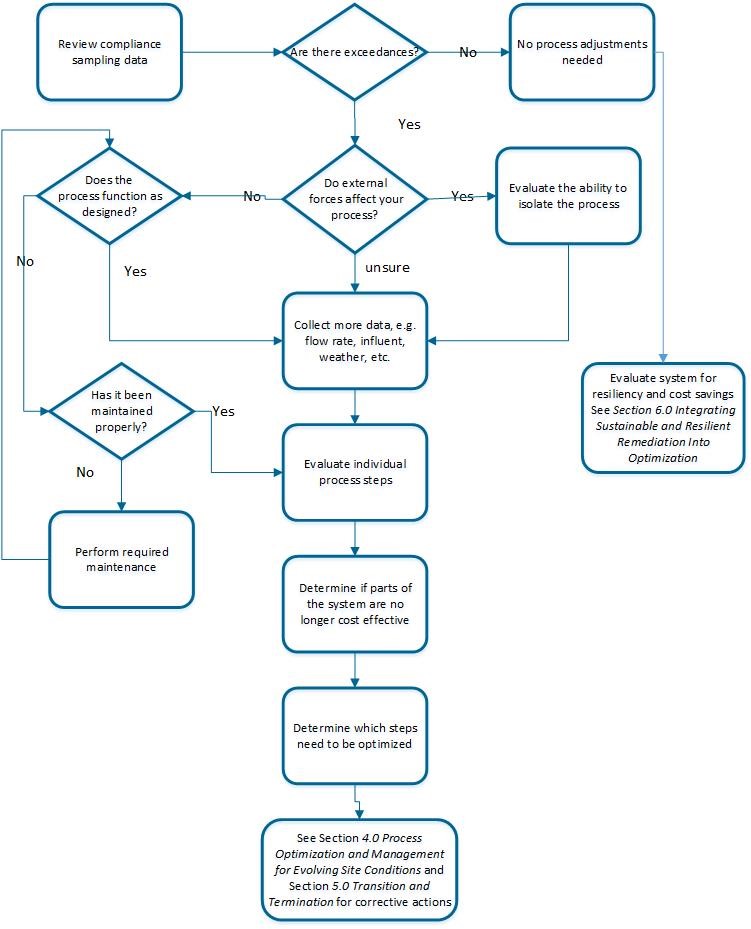
Source: P. Locklin. Used with permission.
3.3.6 Evaluation of Conveyance System and Other Infrastructure
Many P&T systems have been operating for several decades. Aging infrastructure may contribute to the substandard performance of a P&T system or create difficulties in collecting the monitoring data required to complete the performance evaluation process. The infrastructure directly affecting the system performance may include obsolete or inefficient treatment system units and equipment; deteriorated extraction wells, pumps, conveyance piping, and electrical equipment; and inadequate controls. Data collection may be hindered by obsolete or inadequate transducers, flow meters, and samplers, among other things. Frequently, elements of the aging infrastructure are not compatible with newer equipment, or the replacement parts are difficult to obtain or have been discontinued. Therefore, a review of the infrastructure-related issues should form part of the overall system performance evaluation. The review should include assessing the costs and benefits of replacing the aging infrastructure. Factors to be considered include the anticipated life cycle of the system, effect of the investment on improving the system performance, energy efficiency, carbon footprint, and electrical/building codes. Section 4.3.3.5 discusses modifications and improvements to the conveyance system. Sections 6.1.5, 6.5, and 6.7 discuss building sustainability and resiliency into P&T systems.
Appendix B includes case studies for the Lawrence Aviation Industries site in New York and DOD Installation 2 (a landfill CERCLA site) that highlight evaluations of the treatment and conveyance system. These evaluations supported actions, including adjustments and operational changes.
3.4 Is P&T Still the Best Remedial Option for Cleanup?
The findings developed as part of the evaluation process described in this section should be summarized in a P&T evaluation document (see the Groveland case study and report, ( USEPA and USACE 2002[JTCTFQ3A] USEPA, and USACE. 2002. “Remediation System Evaluation, Groveland Wells Superfund Site.” Office of Solid Waste and Emergency Response. https://www.epa.gov/sites/default/files/2015-06/documents/final_groveland_rse_093002.pdf. )). The evaluation findings and recommendations contained in the evaluation document should be sufficient to support decisions regarding the future use and effectiveness of the P&T system. The decision will be based on these key factors:
- ability of the system to meet the updated project objectives (e.g., protection of receptors, plume containment, attainment of discharge standards after treatment, energy efficiency, project timeline)
- cost-effectiveness compared to other technologies
- acceptance by regulators and the project stakeholders
- technical improvements
- achievement of site completion/closure and reuse, where applicable
If the performance evaluation indicates that the project objectives are being achieved in a cost-effective manner, and the approach is acceptable to the regulators and project stakeholders, little or no further modifications to the system are required. If the performance assessment indicates that a P&T system does not satisfy the requirements, the system can be optimized to improve its performance, a different technology can be evaluated and put into place, or the system operation can be terminated. Section 4, Process Optimization, provides a discussion of how to optimize the system to improve performance. Optimization may also address a transitional function, one that is supportive of a repurposed role for the P&T system (e.g., hydraulic control/containment system for alternative in situ treatment methods or extraction and treatment of emerging contaminants not previously within the regulatory or operational objectives). If the findings conclude that a system is beyond rehabilitation, modification/improvement, or repurposing to meet refined stakeholder-shared objectives, Section 5 (Transition and Termination) of this guidance document is relevant. That section provides considerations and steps to identify appropriate transition strategies to ultimately decommission the P&T system.
Key Takeaways Performance evaluations are an iterative process. They are not “one-and-done.” Periodic evaluations should be included in scheduled maintenance, after milestones in the treatment process, and after external events such as fires and major storms. Evaluations should include checking for resiliency and sustainability. P&T system evaluations may show that the system is already working in an optimal fashion (see the Saco Defense, Inc., case study). The evaluation may also show that system optimization is warranted, or that P&T is no longer effective and the remedy should be transitioned to other technologies. |